Manufacturing Chemist, 2022
When it comes to measuring the cytotoxicity of CAR-T cells, not all cell viability assays are created equal, says Daniel Millard, Applications Director at Axion Biosystems
Adoptive cell therapies have broken new boundaries in cancer treatment, but researchers and manufacturers still have a long way to go. These therapies are only approved to treat approximately 20 cancer types and the proportion of patients who exhibit a durable response to treatment is fewer than one in five.1,2
Furthermore, adoptive cell therapies can produce debilitating and sometimes lethal side-effects.3,4 As scientists work to develop safer, more effective immunotherapies, cell viability assays are essential to accurately characterise cellular interactions in vitro and ensure consistency in cell-based products.
The manufacturing process for adoptive cell therapies is complex and involves a variety of biological, logistical and patient safety considerations. The key to manufacturing safe and effective adoptive cell therapies is testing them against in vitro cancer cells as a model.
Researchers have a handful of conventional options available to test cell viability; but, as cell therapy development matures, some seek more sophisticated, safer and easier-to-use techniques that directly inform scientists about a therapy's cytotoxicity. Below are five approaches to measuring cell viability that demonstrate the challenges and opportunities associated with measuring the quality of adoptive cell therapies.
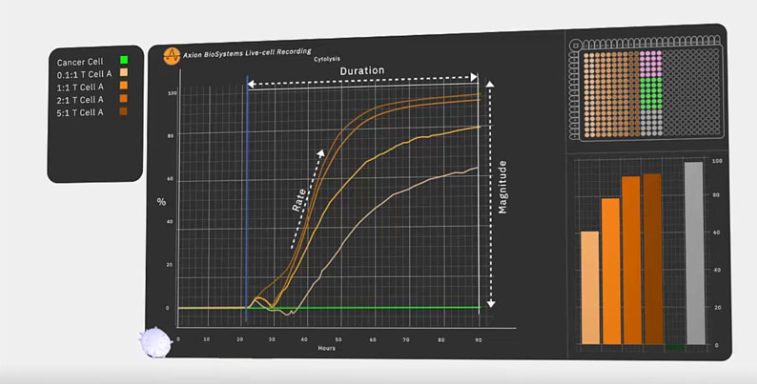
Chromium release assays: Since 1968, scientists have used chromium release assays to study the cytotoxicity of various compounds and other factors. It is still a widely accepted method to measure the potency of adoptive cell therapies.
The assay is straightforward: researchers insert a radioactive isotope of chromium into target (tumour) cells and then apply active CAR-T or CAR-NK cells. As the target cells die, they leak radiation. After a certain number of hours, researchers measure the amount of radiation emitted by the sample. The strength of the radioactive signal indicates the magnitude of cell death.
Many researchers depend on chromium release assays; some, however, seek a new approach with fewer limitations, such as endpoint assays. These only measure cell death at the end of the interaction, blinding them to the nuances of adoptive cell therapy kinetics.
This limitation prevents users from assessing metrics such as peak killing rate, as well as signs of chronic activation and T-cell exhaustion. Furthermore, this test uses toxic radiation as an indicator. Fortunately, researchers have identified safer methods to measure cell viability in the past few decades.
MTT assays measure cell death using a non-toxic indicator, the tetrazolium salt MTT. Mitochondria contain an enzyme that transforms MTT into purple crystals. Once cells die, this reaction stops. Using a spectrophotometer, researchers can quantify the concentration of purple colour, which represents the extent of cell death — the less intense the purple colour, the higher proportion of dead cells in the sample.
MTT assays are cheap and easy to perform. And, as they don’t release harmful radiation, they are safe. But they share one important limitation with chromium release assays: MTT assays are also endpoint assays. Consequently, researchers cannot measure the kinetics of cytotoxicity.
LDH assays: All cells produce lactate dehydrogenase (LDH), which leaks out when a cell membrane gets damaged. Hence, LDH can serve as an indicator of cell death in a dish. But researchers cannot measure LDH directly, which complicates the assay.
First, LDH converts lactate into pyruvate. When pyruvate interacts with a tetrazolium salt in the extracellular solution, it forms a water-soluble red molecule. Researchers then quantify the red colour’s intensity using colorimetry; the more intense the red colour, the more potent the therapy. Like the first two examples, LDH assays are also endpoint ones. However, new generations of cell viability assays have solved this problem.
Live-cell imaging is an optical technique that scientists can use to visualise adoptive cell therapy activity. Unlike all the methods mentioned above, live-cell imaging enables researchers to measure cell death in real-time. Researchers can measure cell-killing continuously from hours to weeks and detect critical events throughout the experiment.
Although live-cell imaging overcomes one of the primary limitations of traditional cell viability assays, this technique introduces new challenges. To conduct a live-cell imaging experiment, users plate tumour cells before adding a layer of engineered immune cells and watching them interact.
The engineered cells may block the view of the tumour cells, making it difficult to see when the tumour cells die. Some scientists use dyes to differentiate the two cell types to overcome this limitation, but this extra manipulation threatens the study’s reliability and validity. Furthermore, this method produces visual data that researchers must analyse subjectively, creating uncertainty in the results.
Impedance monitoring with a bioelectronic assay: Engineers built bioelectronic assays to overcome some of the limitations of earlier cell viability assays. These assays allow scientists to non-invasively detect cell death in real-time without using a microscope or any probes or dyes. As such, these assays can continuously monitor complex cellular interactions without the interference of chemicals from hours to weeks.
Bioelectronic assays take place in multiwell plates containing bioelectronic sensors at the bottom of each well. To test the effect of cancer treatments, researchers apply a layer of tumour cells to the plate, and these cells attach to the electrodes.
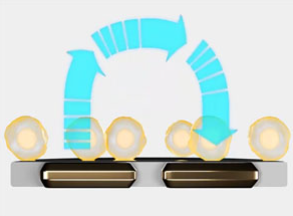
Next, the researchers add a layer of immune cells and monitor the interaction. The tumour cells, which initially impede the electrical signal emitted from the electrode, die. When this happens, they detach and the recorded impedance drops. As impedance correlates directly with cell viability, researchers can observe the kinetics of cell death with time. Furthermore, researchers can test and compare multiple doses at one time using the multiwell plate.
Dr Lohitash Karumbaiah, an Associate Professor at the University of Georgia, used a bioelectronic assay to assess the potency of a novel CAR-T cell therapy designed to kill glioblastoma cells.5 Dr Karumbaiah found that his CAR-T cells killed a significantly higher proportion of glioblastoma cells than naive T cells during a 7 day period.
The bioelectronic assay was sensitive enough to measure cell death in conditions featuring a low concentration of CAR-T cells. It also measured the impact of multiple CAR-T cell concentrations at once. Overall, the bioelectronic assay enabled Dr Karumbaiah to gain more insights into GD2-targeting CAR-T cells as a potential therapeutic option for people with glioblastoma.
Measuring cell viability to assess immune cell potency
An adoptive cell therapy's potency is a key measurement during development and manufacturing. Researchers need an accurate understanding of a treatment’s ability to perform as expected and drug makers must ensure the consistency of cell-based products from batch to batch.
Researchers have used traditional cell viability assays for decades. Still, as adoptive cell therapies become more commonplace, researchers will benefit from using more sophisticated assays that provide more information about treatments earlier in the development process.
Bioelectronic assays are the latest generation of cell viability assays. They enable researchers to assess the kinetics of adoptive cell therapies in real-time without using dyes, labels or probes that could interfere with biological activity. A bioelectronic assay is a biopharmaceutical manufacturer’s best option to accurately characterise cellular interactions and ensure product consistency.
References
- www.cancerresearch.org/en-us/join-the-cause/cancer-immunotherapy-month/30-facts/04.
- www.hopkinsmedicine.org/inhealth/about-us/immunotherapy-precision-medicine-action-policy-brief.html.
- www.cancer.net/navigating-cancer-care/how-cancer-treated/immunotherapy-and-vaccines/side-effects-immunotherapy.
- https://my.clevelandclinic.org/health/diseases/22700-cytokine-release-syndrome.
- https://aacrjournals.org/cancerres/article/81/13_Supplement/1552/667462/Abstract-1552-Kinetics-and-potency-of-T-cell-and.