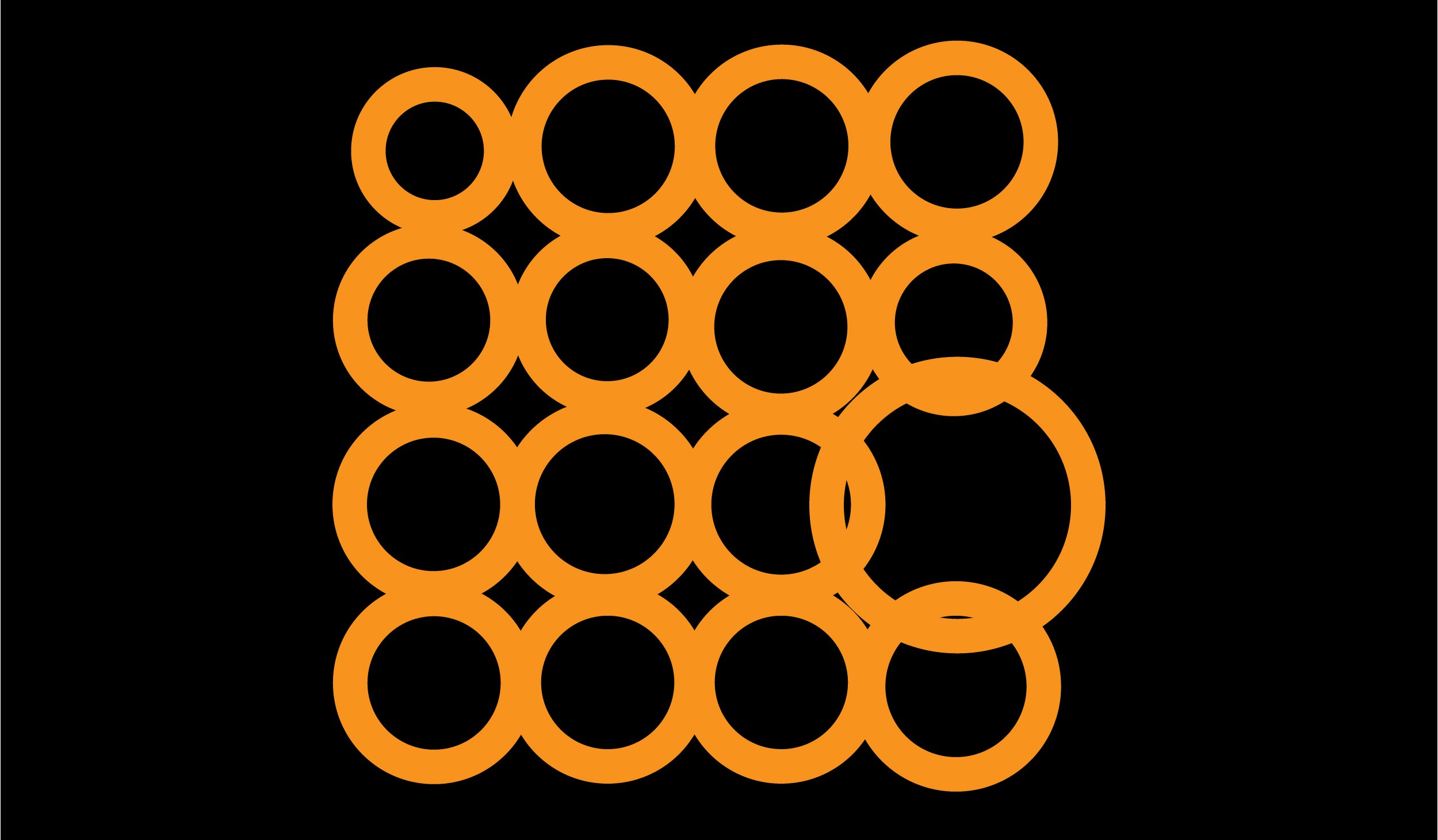
心筋細胞の収縮力は、変力作用によって増減します。陽性変力作用は心筋の収縮力を増大させ、陰性変力作用は減少させます。これらの陽陰性変力作用は、心臓血管系の活動において重要な役割を担います。
MEAプレート上で培養された心筋細胞は、自発的な拍動を有し、電極上で弛緩収縮の動きを繰り返します。Maestro Pro/Edgeの [Contractility] 機能は、この弛緩収縮による心筋細胞の形状の変化を、電極との接着面におけるインピーダンスの変化としてとらえます。
Maestro Pro/Edgeは、同一電極から、細胞外電位測定とインピーダンス変化の両測定が可能です。電気的な活動と弛緩収縮の両評価により、心筋細胞の機能をより深く理解することができます。
Force-frequency 応答による心筋細胞成熟化の検証
ヒトiPS細胞由来心筋細胞 (hiPSC-CMs) は、カルシウムハンドリング、収縮・弛緩等の機能においてその未成熟さが議論されています。一方で、電気的或いは機械的な刺激は、細胞を成熟化させる⼿段の1つとして有効性が⽰されています。本事例では、Maestro MEAを用いて48時間の連続ペーシングを行い、成熟の指標の1つとされる Force-frequency relationship を検証しました。
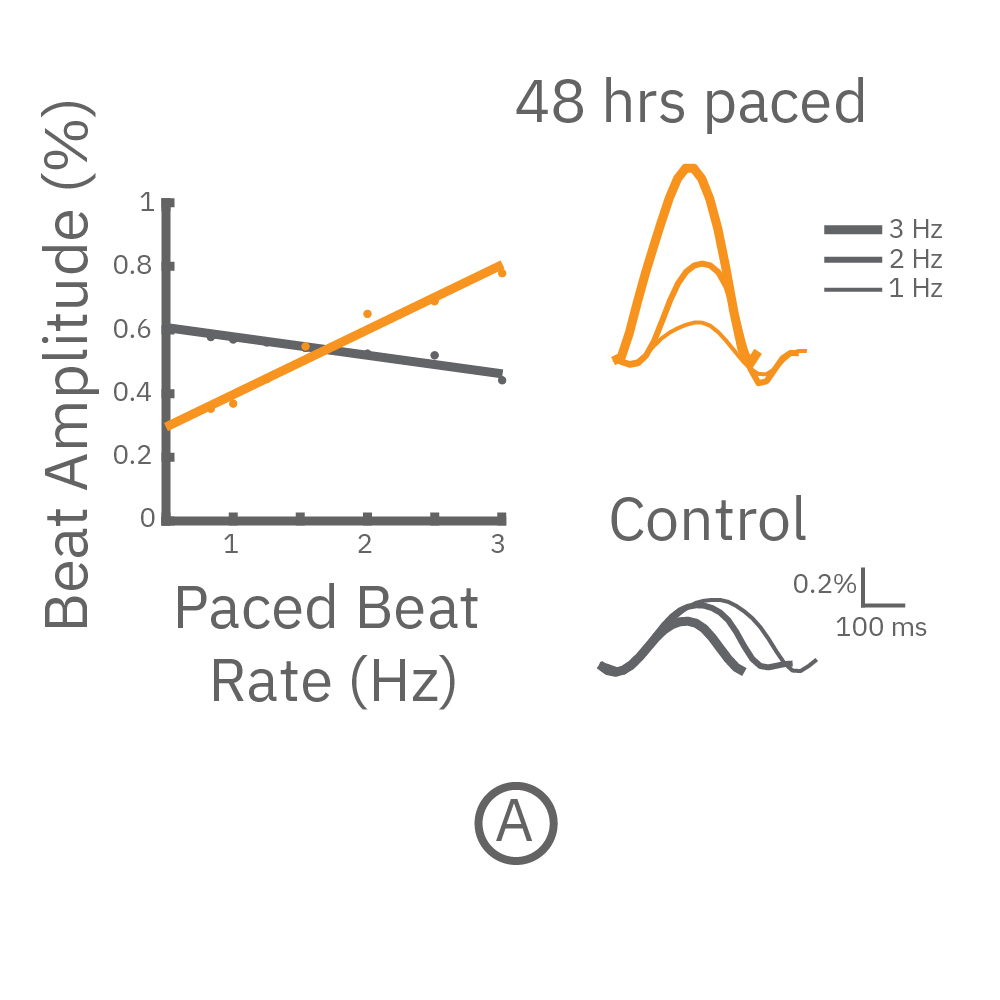
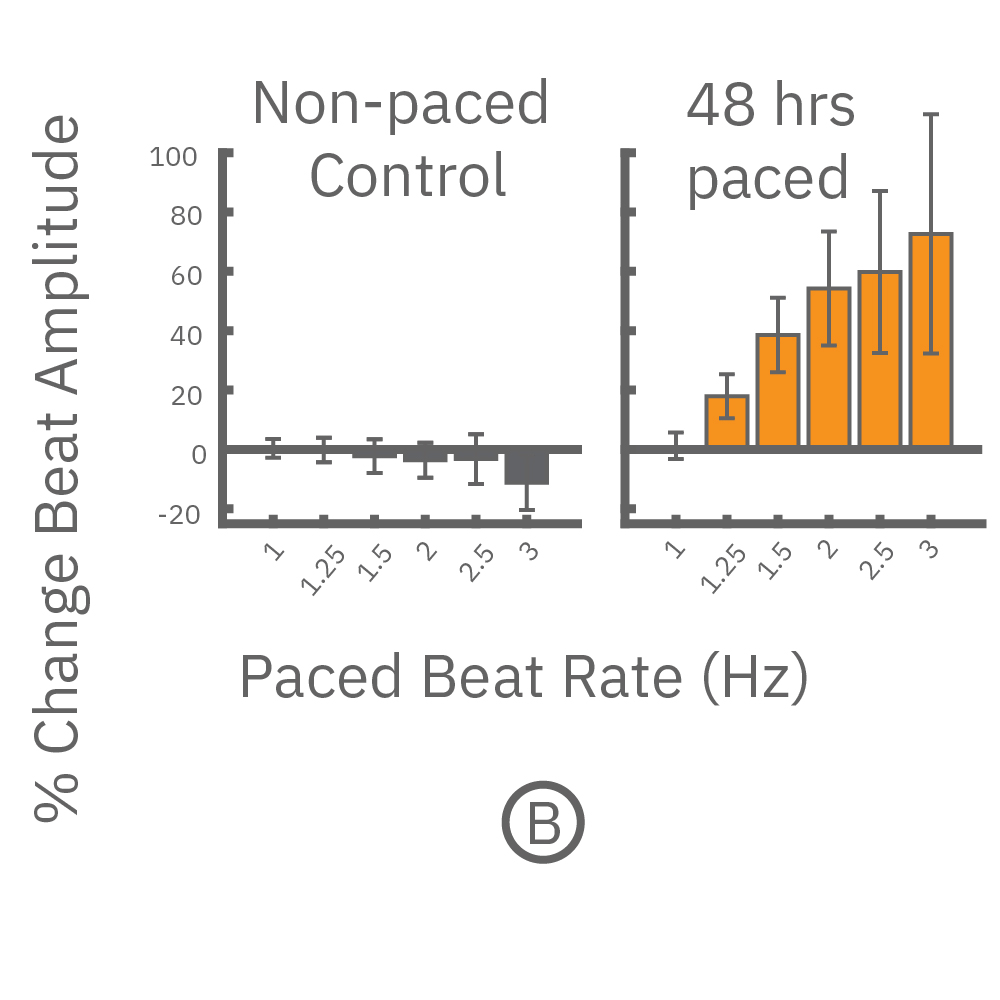
(A) CytoView 24 well MEA プレート上に iCell CM² (富士フィルムCDI)を培養し、⼀定の成熟後(DIV7-10) に2Hzのペーシングを48時間連続して印加した。ペーシング終了後、1〜3Hzのペーシングを連続印加しForce-frequency relationship を検証した。48時間連続ペーシングした iCell CM²では、刺激頻度の上昇と共に、拍動振幅値 (Beat Amplitude) の上昇が得られた (オレンジ色)。⼀⽅、Control (連続ペーシング無) の細胞では拍動振幅値は減少した(グレー色) 。右図は、1Hz、2Hz、3Hzペーシング印加時の波形を示す。
(B) 各刺激頻度毎の 拍動振幅値の変化を示す。連続ペーシングを印加した心筋細胞では、拍動振幅値の上昇が得られ(オレンジ色)、ペーシング無しの細胞では、わずかな減少が得られた(グレー色) 。
変力作用化合物の評価
ヒトiPS細胞由来心筋細胞の未成熟な表現型は、変力作用化合物への乏小な反応、特にβアドレナリン受容体アゴニストに対する陽性変力性応答の欠如や減少にて示されます。本事例では、Maestro MEAで2Hzのペーシングを48時間連続して行い、陽性変力化合物の評価を行いました。
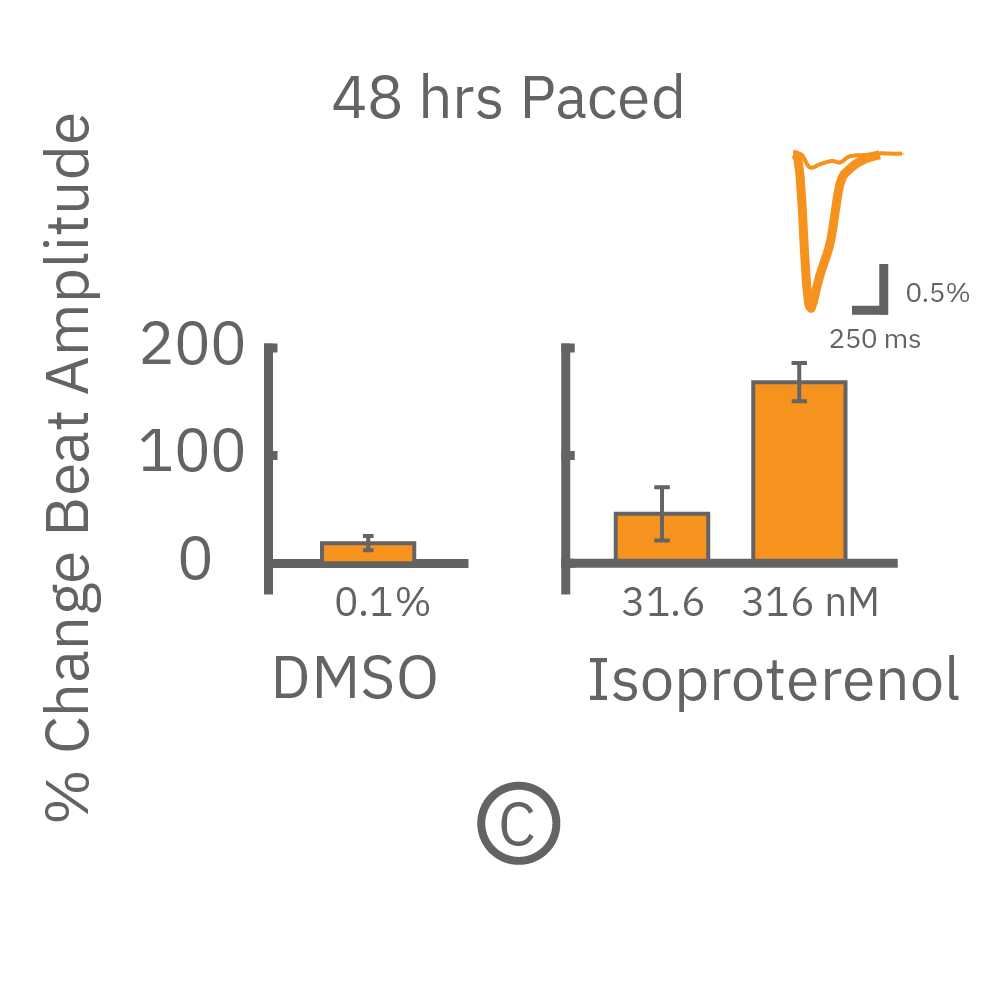
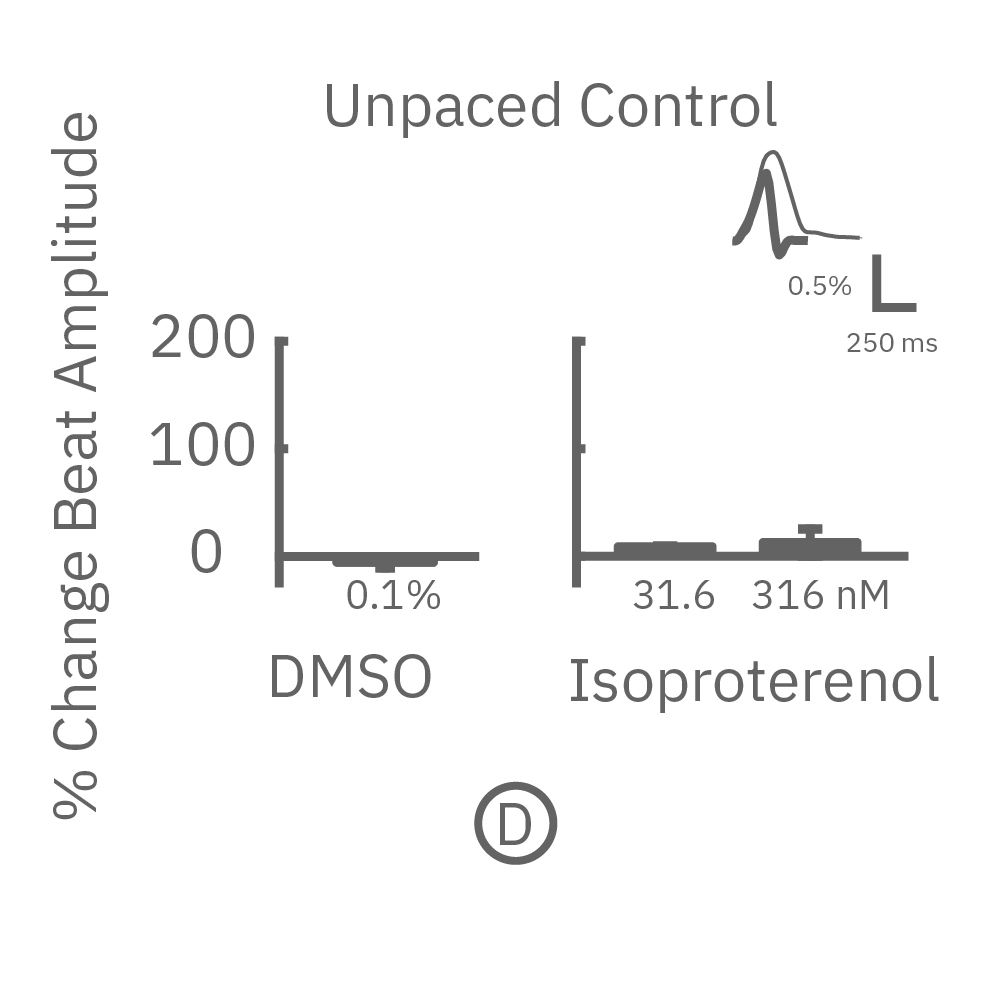
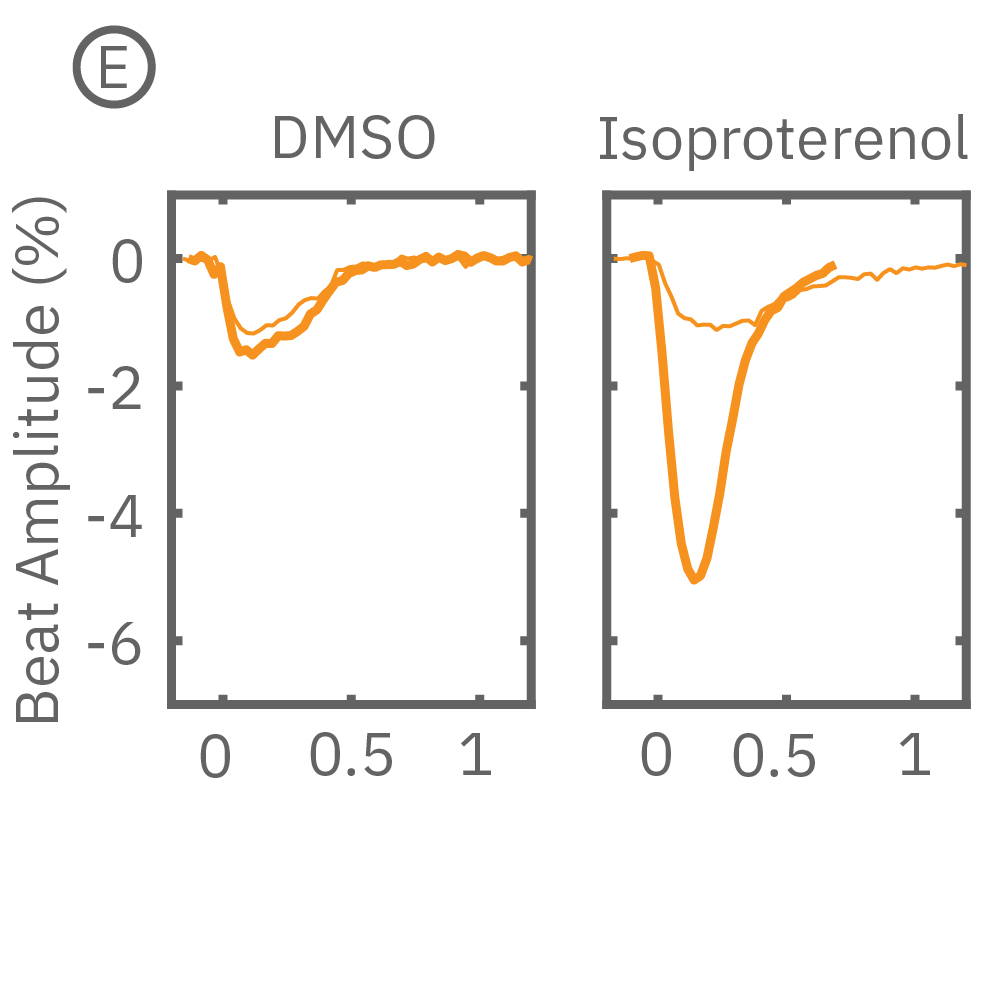
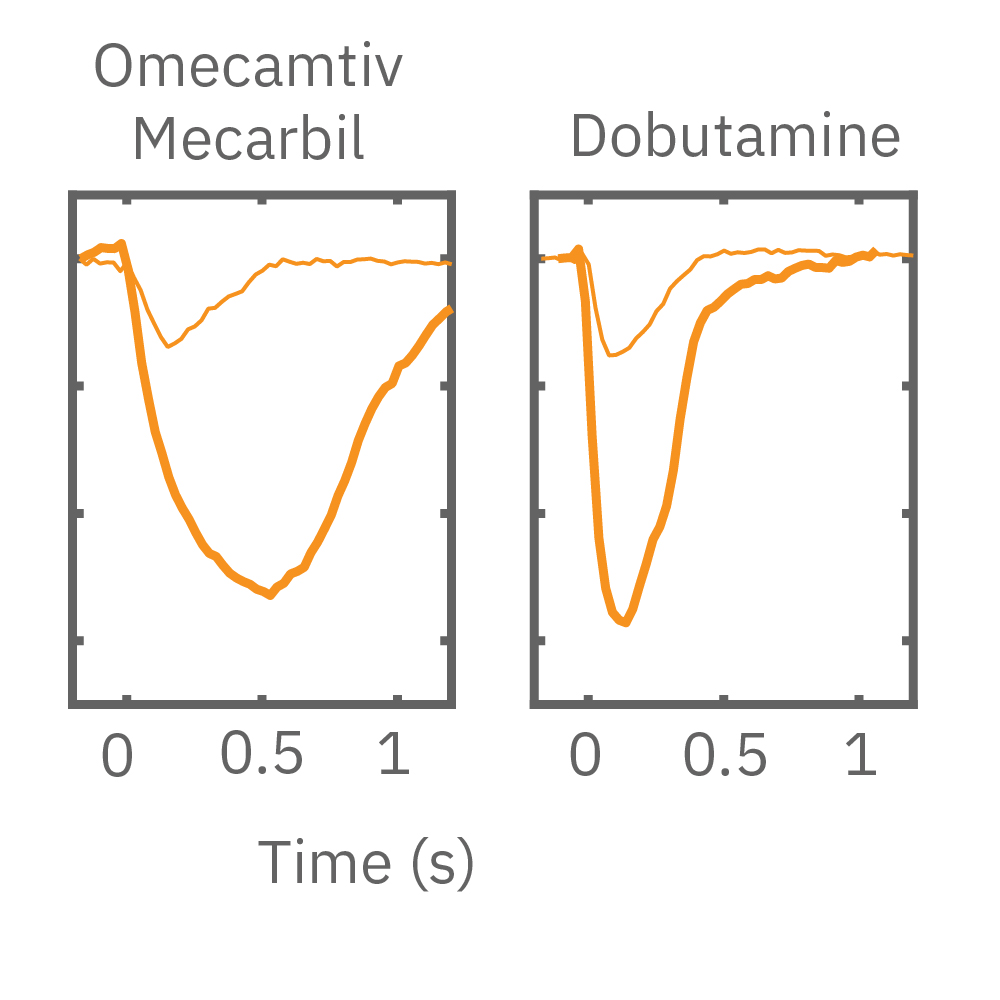
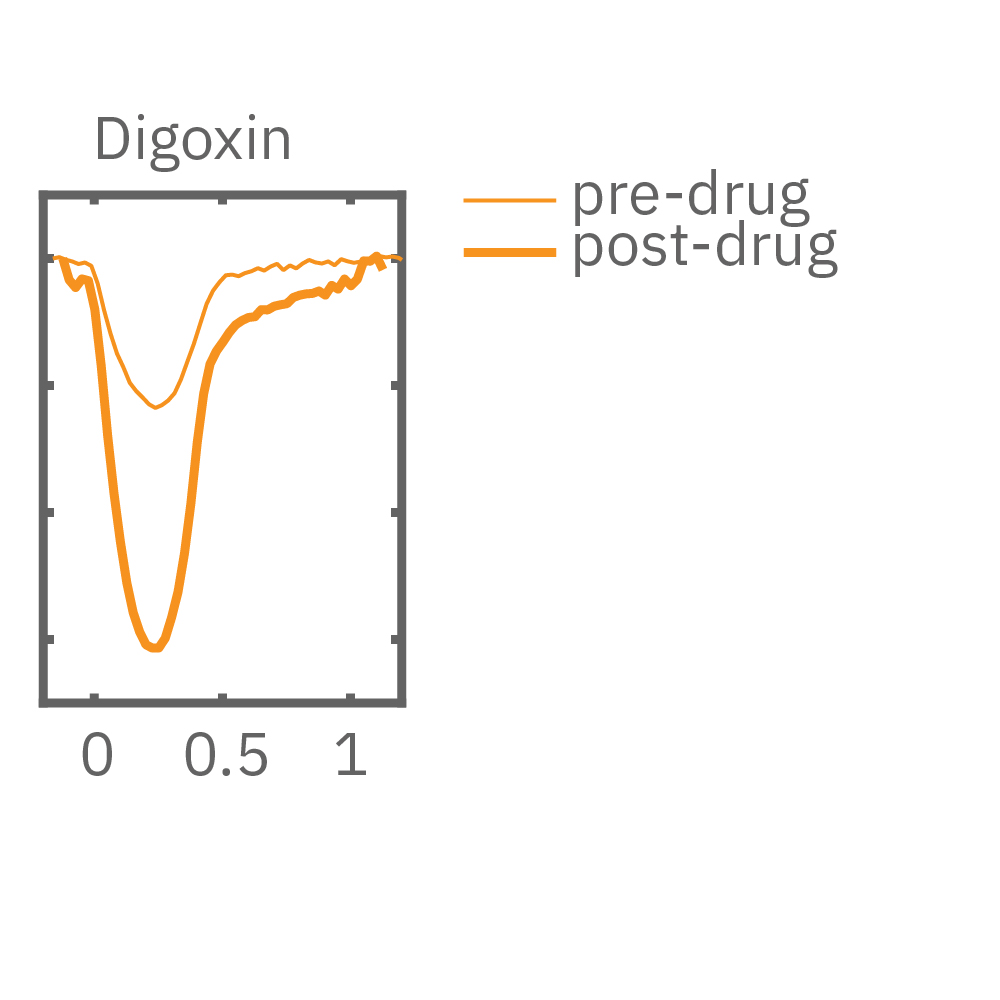
MEA プレート上に培養されたiCell CM² (富士フィルムCDI) に48時間連続して2Hzのペーシングを行った後、陽性変力作用薬(イソプレテレノールなど)を投与した。
(C) 48hペーシングを印加した心筋細胞の拍動振幅値の変化率を示す。イソプレテレノール (βアドレナリン受容体アゴニスト) の投与により、拍動振幅値の上昇が得られた。
(D) 48hペーシング非印加(コントロール)の心筋細胞の拍動振幅値の変化を示す。DMSO、イソプレテレノール共に顕著な変化は得られなかった。
(E) 48hペーシング印加した心筋細胞へのDMSO及び複数種類の陽性変力作用薬投与時の拍動波形の変化を示す。全ての変力作用薬に対して、拍動振幅値の上昇が得られた。
3D培養における収縮変化の検証
スフェロイド、オルガノイドなどの in vitro の3D 細胞モデルは、in vivo 組織の多層構造をより正確に再現することが可能であることから、疾患モデリング、発生生物学、安全性試験などにおいて、その有用性が提起されています。本事例では、MEAプレート上に播種された複数の3Dスフェロイドから Contractility (インピーダンス変化)を測定しました。
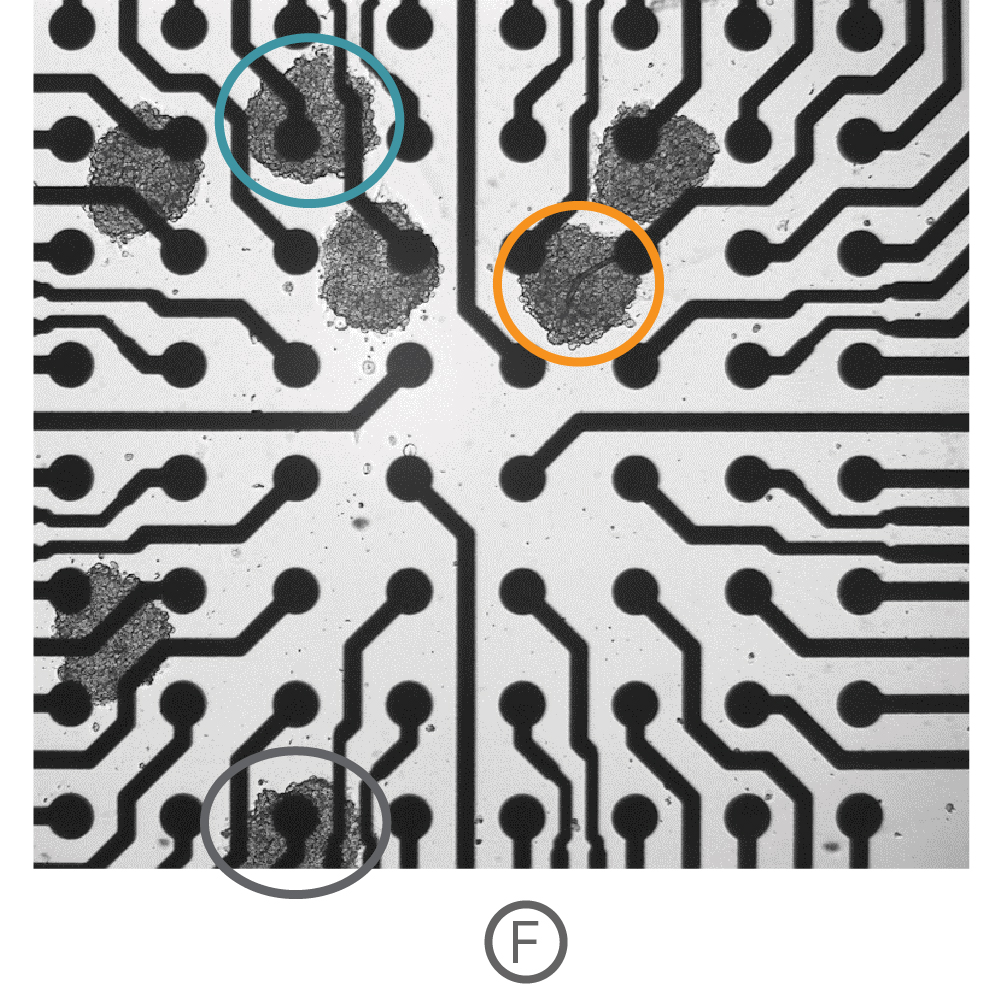
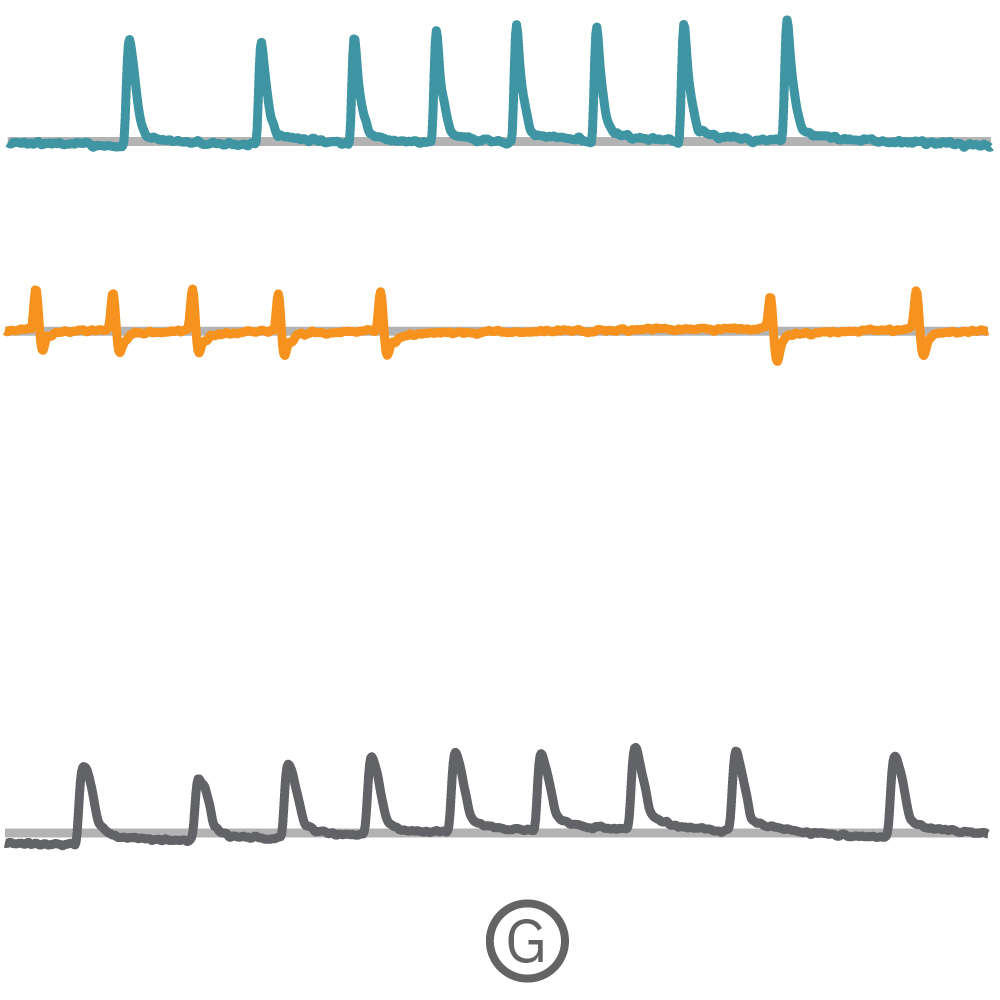
(F)複数の心筋細胞スフェロイド (Ncardia, Cor.4U) をCytoView 6well MEA プレート上に播種し、電極への接着後、測定を行った。
(G) Fで示された各電極から取得された Contractility波形を示す。
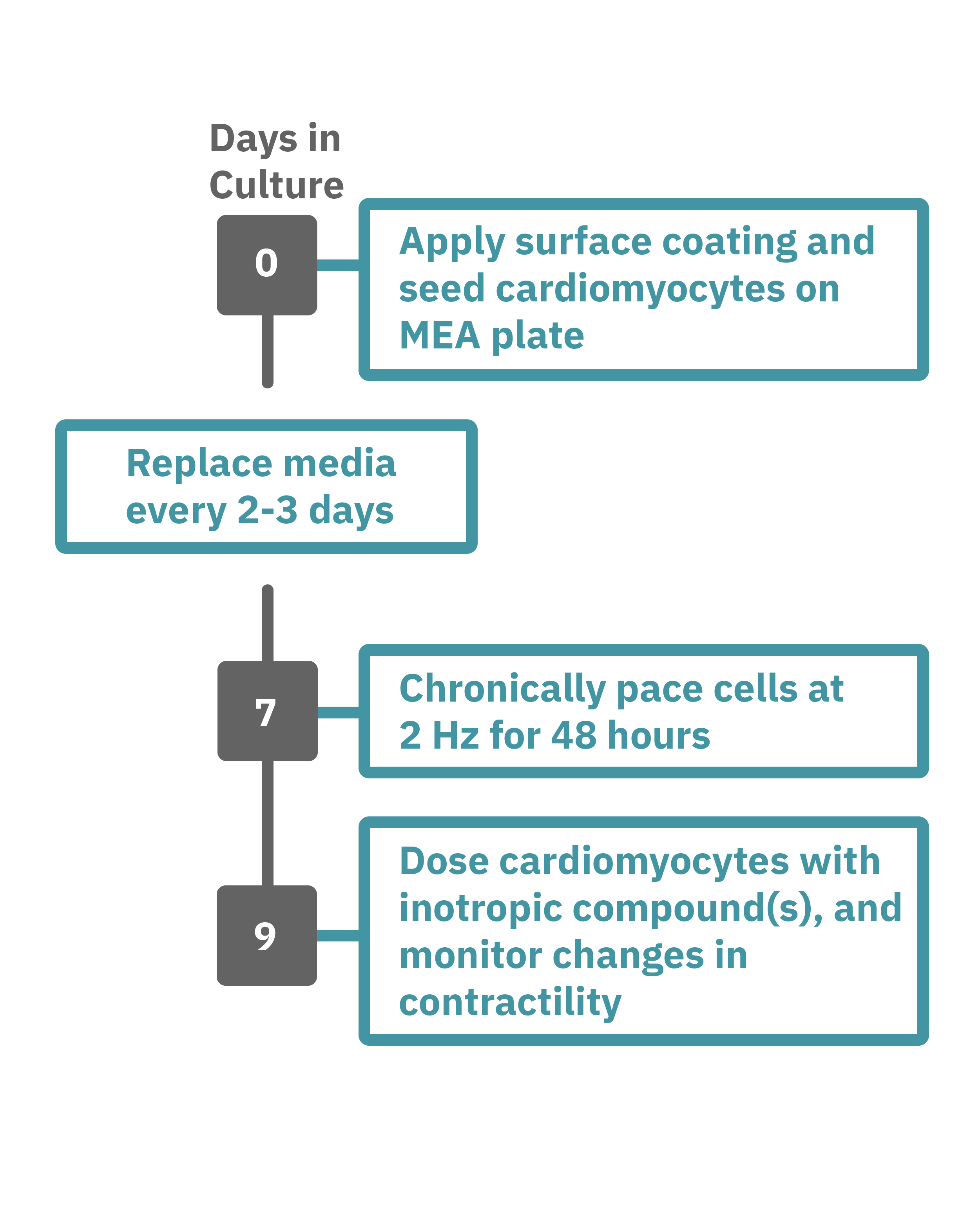
Maestro Pro/Edge による心筋細胞弛緩収縮評価は非常に簡単です。
コーティングされたMEAプレート上に直接心筋細胞を播種します(Day 0)。2-3日毎に培地交換をしながら、細胞を培養します。
一定の成熟が得られた後 (例: Day 7+)、プレートを Maestro に搭載し2Hzのペーシングを48時間に渡り連続して印加します。48時間後、ペーシングを停止し、変力作用薬などを投与してデータ測定を行います。データは付属のソフトで解析可能です。
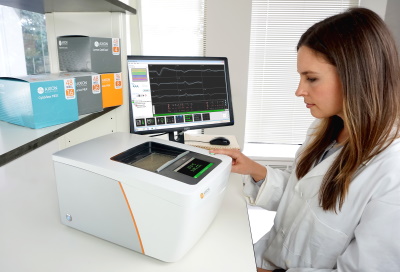
Maestro MEA による心筋細胞弛緩収縮評価:特徴
-
48時間ペーシングによるiPS細胞由来心筋細胞の変力作用評価 - 電気的な刺激は、iPS細胞由来心筋細胞を成熟化させる⼿段の1つとして有効性は⽰されていますが、現在の手法では、成熟の指標の1つとされる Force-frequency relationship (FFR) を得るのに数週間を要します。Maestro MEAによるペーシングでは、48時間の連続刺激でFFRが得られ、変力作用化合物の評価が可能です。
-
1システムで4種類のアッセイ - Maestro Pro/Edgeでは、1枚のプレートで次の4種類のアッセイが行えます。[1] 細胞外電位応答 [2] シグナル伝播 [3] Contractility (弛緩収縮評価) [4] LEAP (活動電位形態シグナル)
-
同一プレート上で培養から測定まで - MEAプレート上で直接細胞を培養し、測定します。環境要因による変化を最小限にとどめながら、細胞への負担が少ない状態で、細胞の変化を検出することができます。
-
ラベルフリー・リアルタイムで細胞の電気的な活動を測定 - プレート上の電極を用いて心筋細胞の活動電位を測定します。ラベルフリー、リアルタイムな測定は、試薬など2次的要因によるゆがみがなく、細胞の変化をより正確にとらえます。また、数日から数週間に渡る慢性評価にも最適です。
-
安定した環境下での実験 - 温度・CO₂ 濃度は、装置搭載のコントローラで自動制御されます。また、Maestro は外来ノイズ・振動に影響されにくい設計になっています。安定した環境で毎日安心して実験に望めます。
-
簡単操作 - 電気生理未経験の方でも簡単に実験が行えます。MEAプレート上に細胞を培養し、装置に搭載するだけで、心筋細胞の電気的な活動の測定が可能です。付属のソフトウエアパッケージを用いて、数クリックで複数の指標に基づいた解析結果が得られ、結果の作表まで行うことができます。
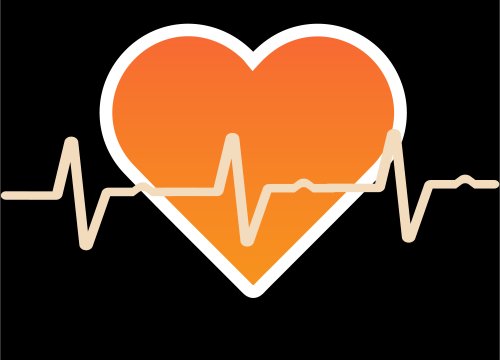
Cardiac MEA
Show Full DetailsWhat is a microelectrode array (MEA)?
Microelectrode arrays (MEA), also known as multielectrode arrays, contain a grid of tightly spaced electrodes embedded in the culture surface of the well. Electrically active cells, such as cardiomyocytes, are cultured on top of the electrodes. When neurons fire action potentials, the electrodes measure the extracellular voltage on a microsecond timescale. As the cells attach and connect with one another, an MEA can simultaneously sample from many locations across the culture to detect propagation and synchronization of cardiac activity across the syncytium.
That’s it, an electrode and your cells. No dyes, no incubation steps, no perfusion, no positioning things just-so; just your cells in a well. Because the electrodes are extracellular (they do not poke into the cells), the recording is noninvasive and does not alter the behavior of the cells, you can measure the activity of your culture for seconds or even months!
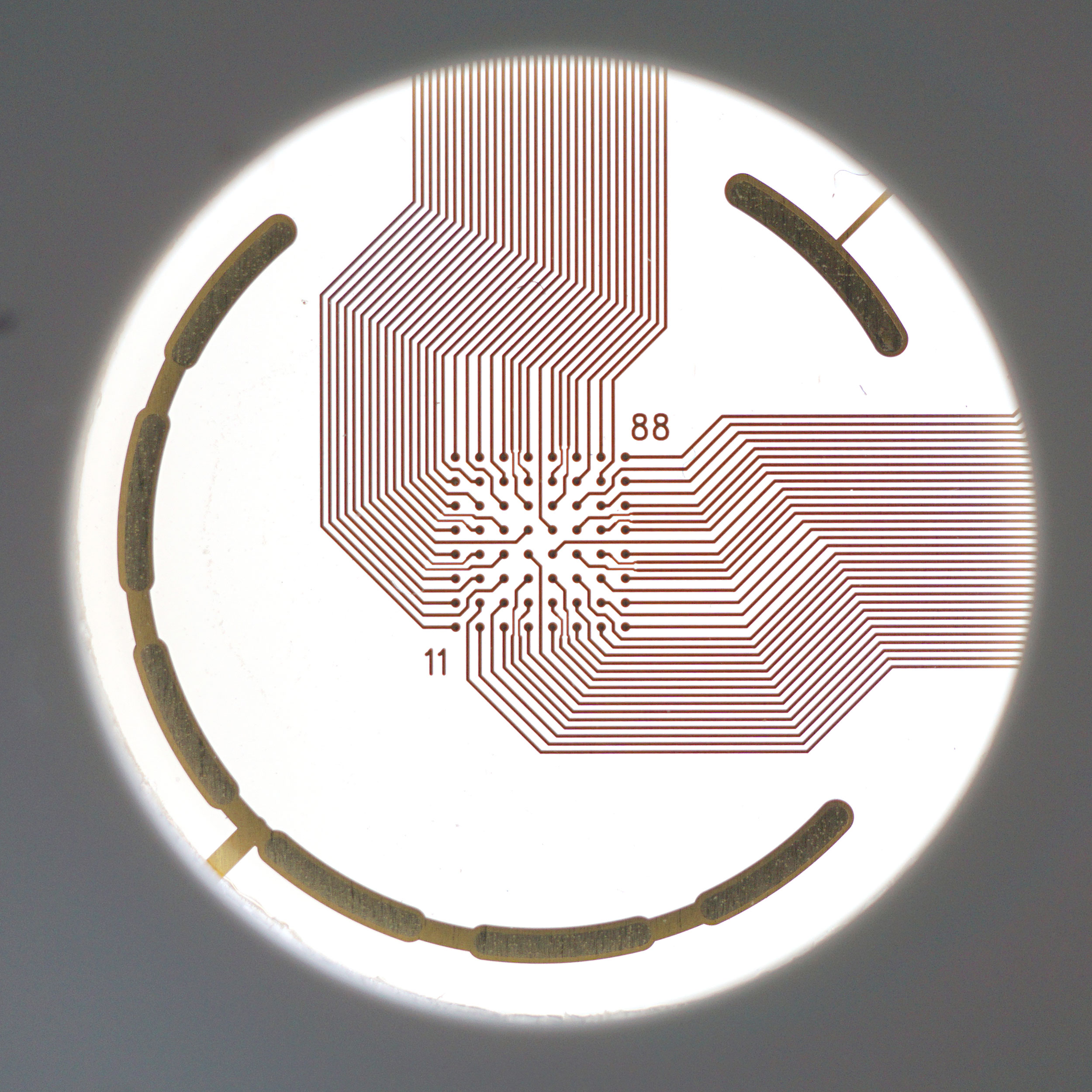
An MEA of 64 electrodes embedded in the substate at the bottom of a well.
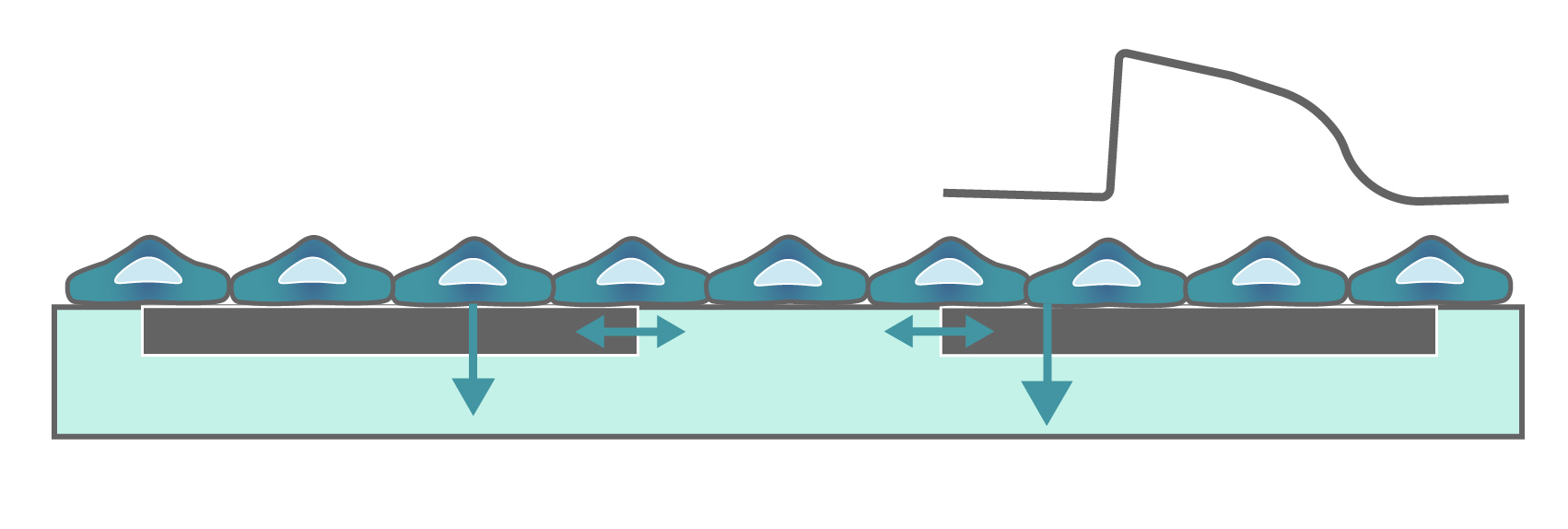
Cardiomyocytes attach to the array and form a network. The microelectrodes detect the action potentials fired as well as their propagation across the network.
Heartbeats in a dish
When cardiomyocytes are cultured on top of an MEA, they attach and connect to form a spontaneously beating sheet of cells, called a syncytium. When one cardiomyocyte fires an action potential, the electrical activity propagates across the syncytium causing each cell to fire and then contract. The electrodes detect each individual action potential and contraction, as well as the propagation of this activity across the array.
The propagating electrical signal is detected by the electrodes as an extracellular field potential. The field potential derives from the underlying cardiac action potential, but more closely resembles a clinical electrocardiogram (ECG) signal. The initial depolarization phase is seen as a sharp spike, similar to the QRS complex, and the slow repolarization is seen as a small slow spike, like a T-wave. The time from the depolarization to repolarization is termed the field potential duration (FPD) and is a key metric in predictive cardiotoxicity screening assays.
While most record the cardiac field potential, the Maestro Pro and Edge MEA systems can also measure local extracellular action potentials, or LEAP. LEAP induction increases the coupling between the microelectrodes and the cardiomyocytes, transforming the extracellular signal from a field potential to an action potential. LEAP provides additional and complementary metrics such as rise time, action potential duration (APD), triangulation, and automated early after depolarization (EAD) detection.
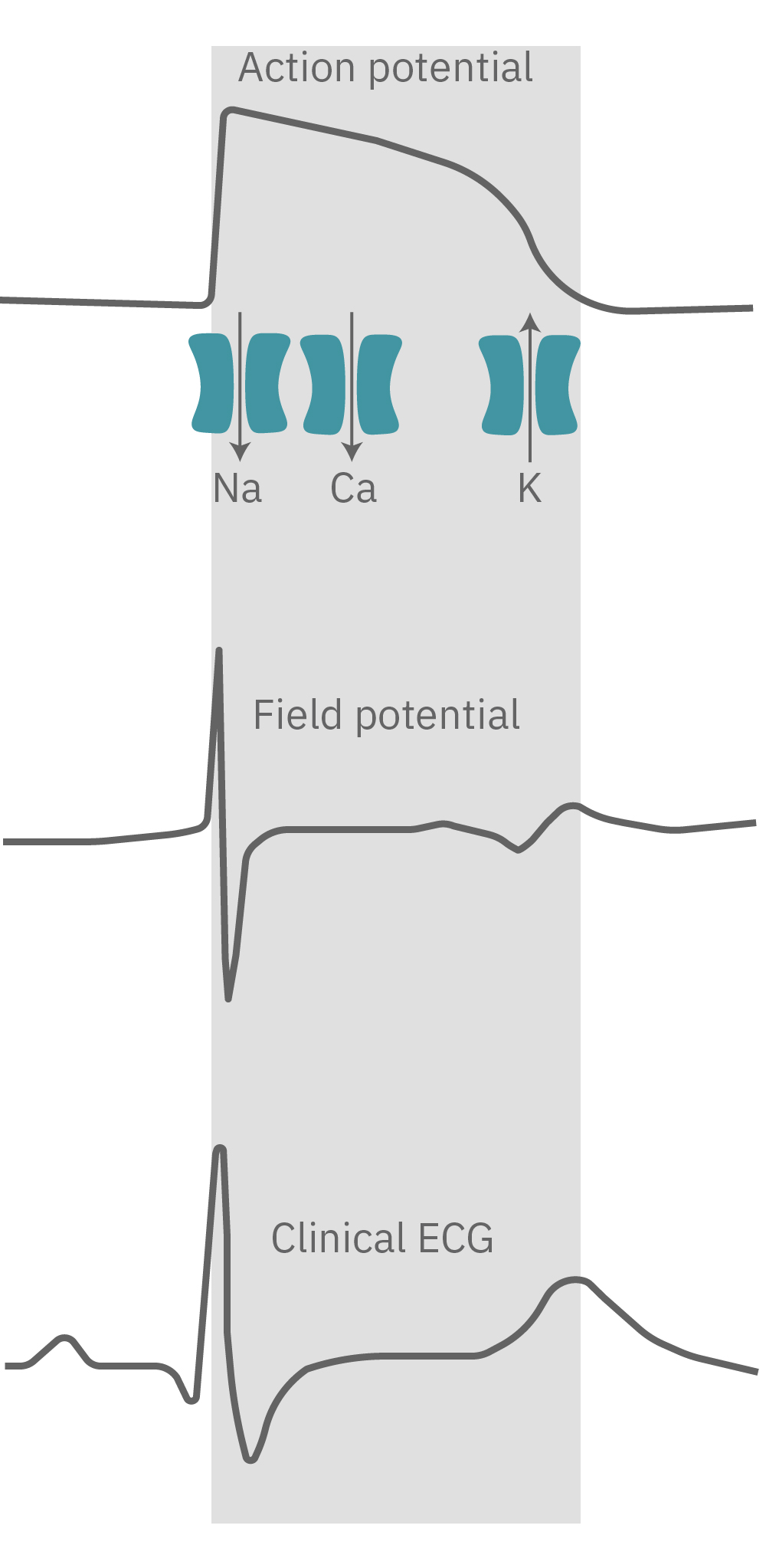
The cardiac action potential propagates from cell to cell across the syncytium. The MEA detects this activity as an extracellular field potential, which closely resembles the clinical ECG.
Do more with multiwell
Axion BioSystems offers multiwell plates at many throughputs, from 6-wells to 96-wells, with an MEA embedded in the bottom of each well. Each well represents its own unique cell culture and conditions, creating up to 96 experiments on one plate. Multiwell MEA allows you to study complex human biology in a dish, from a single cell firing to network activity, across many conditions and cell types at once.
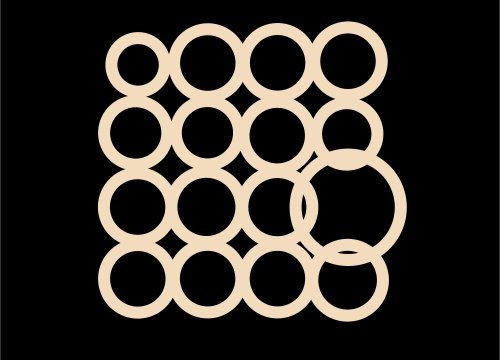
Contractility
Show Full DetailsContractility: Using array-based impedance
Every beat of the heart is characterized by a contraction of the heart that pumps the blood out to the body. When cardiomycoytes are cultured on top of electrodes, they form a spontaneously beating syncytium. With each beat, the cells contract and relax, changing their shape and coverage over the electrodes. These changes can be measured as a change in impedance, or contractility.
Contractility is often used to characterize the mechanical properties of induced pluripotent stem cell-derived cardiomyocytes and to detect the effects of compounds on cardiac contractile function (e.g. inotropes). Measures such as beat amplitude, beat period, and excitation-contraction delay reveal changes in contractile function due to cardiomyocyte maturation or compound addition.
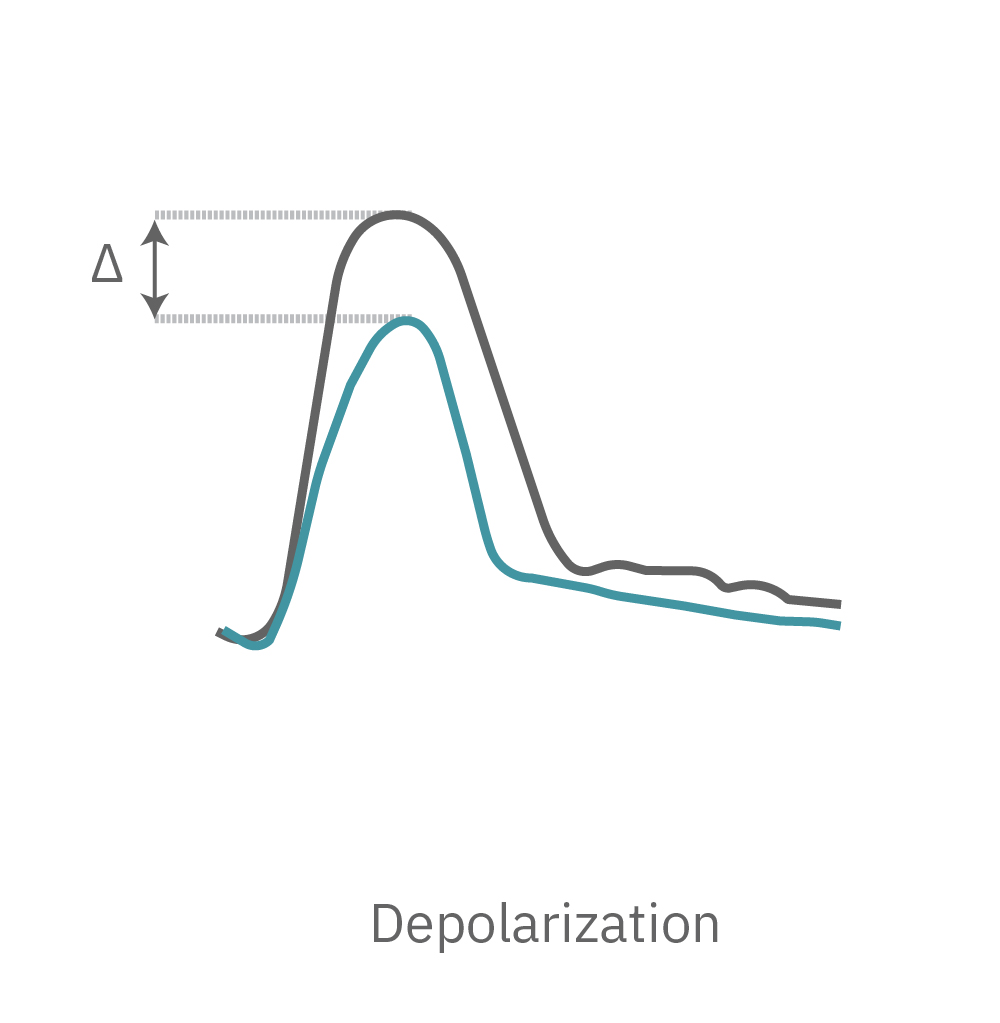
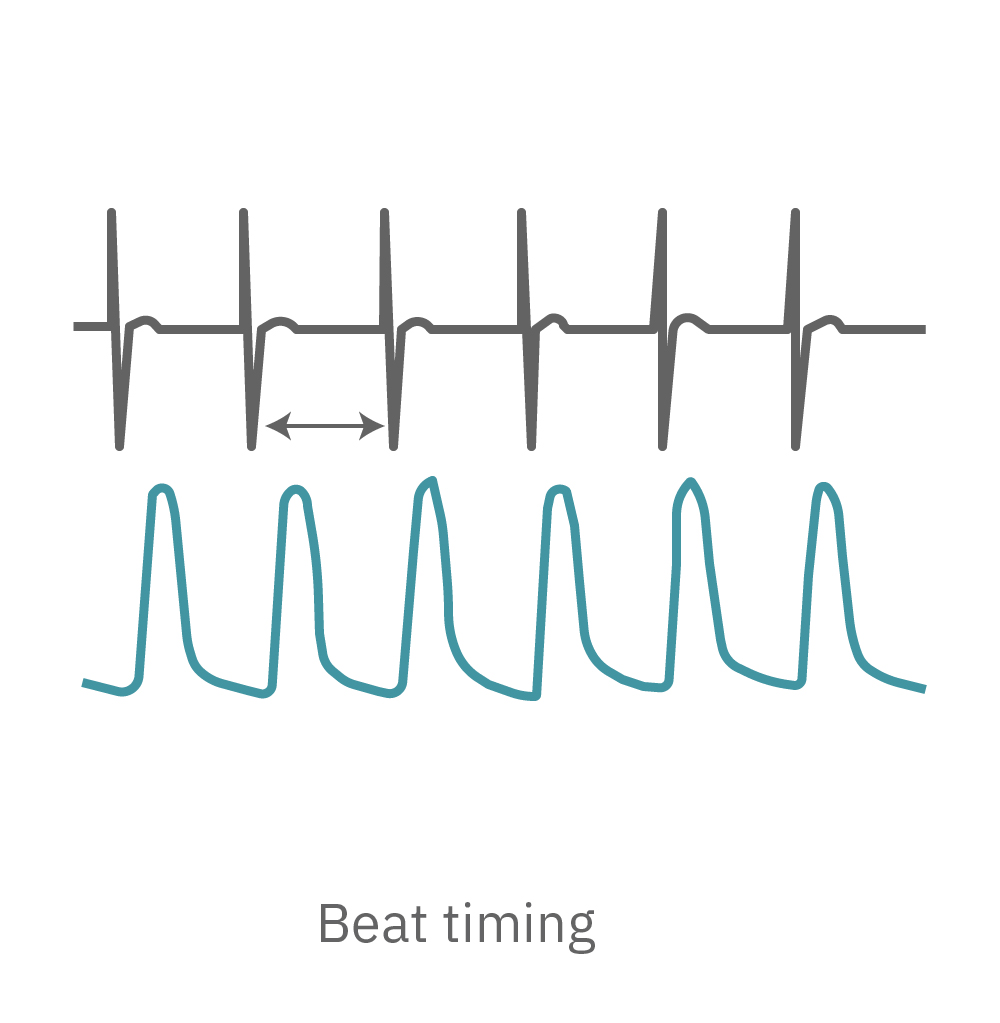
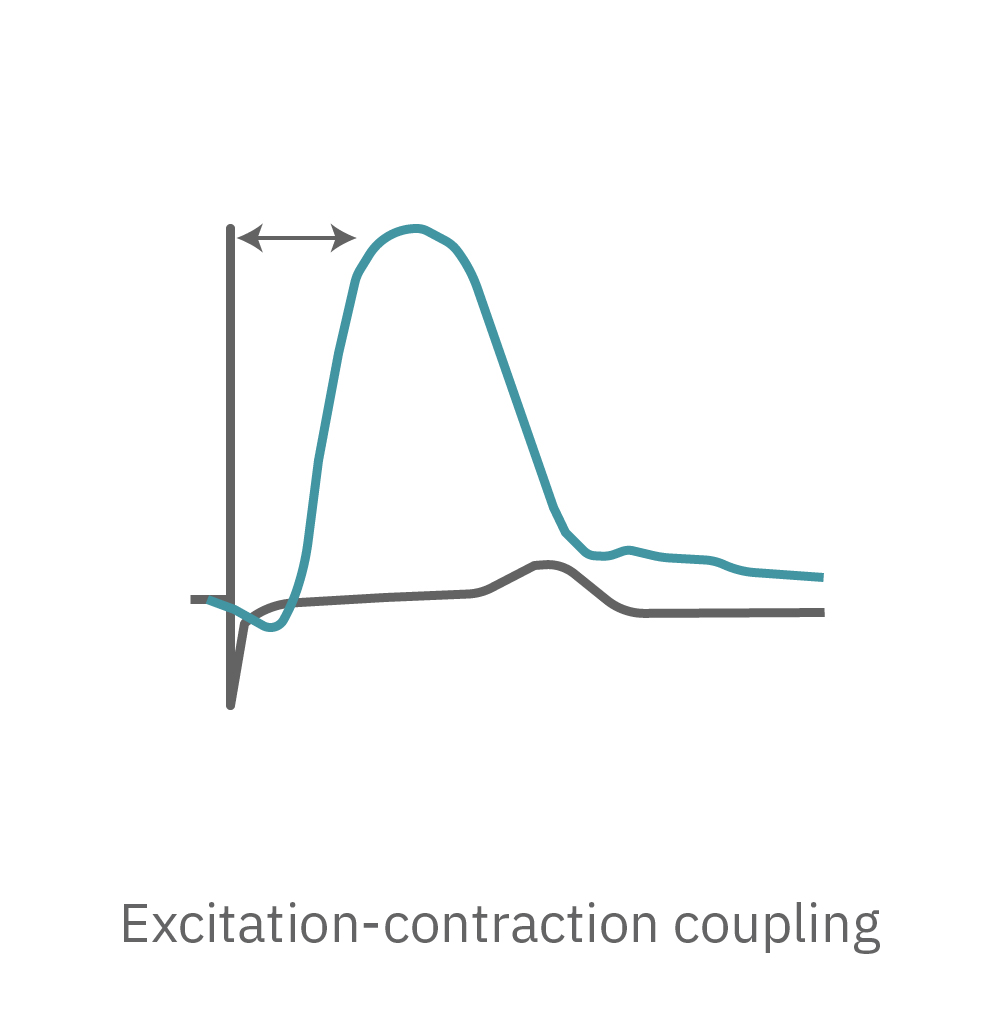
The Maestro Pro and Edge provide key parameters of cardiomyocyte contractile function, including beat amplitude, beat period, and excitation-contraction delay. The Maestro systems are the only platforms that can measure the coupling between the electrical (field potential) and contractile signals from the same cells over the same electrode at the same time.
Most contractility platforms measure contractility from one or two large electrodes. The Maestro Pro and Edge use an array of microelectrodes to measure high-resolution contractility from multiple locations across the syncytium. Array-based contractility can be used to track propagation and characterize variability. In addition, by simultaneously monitoring both the field potential and the contraction from the same electrode, the Maestro provides highly precise measurements of beat timing and excitation-contraction delays.
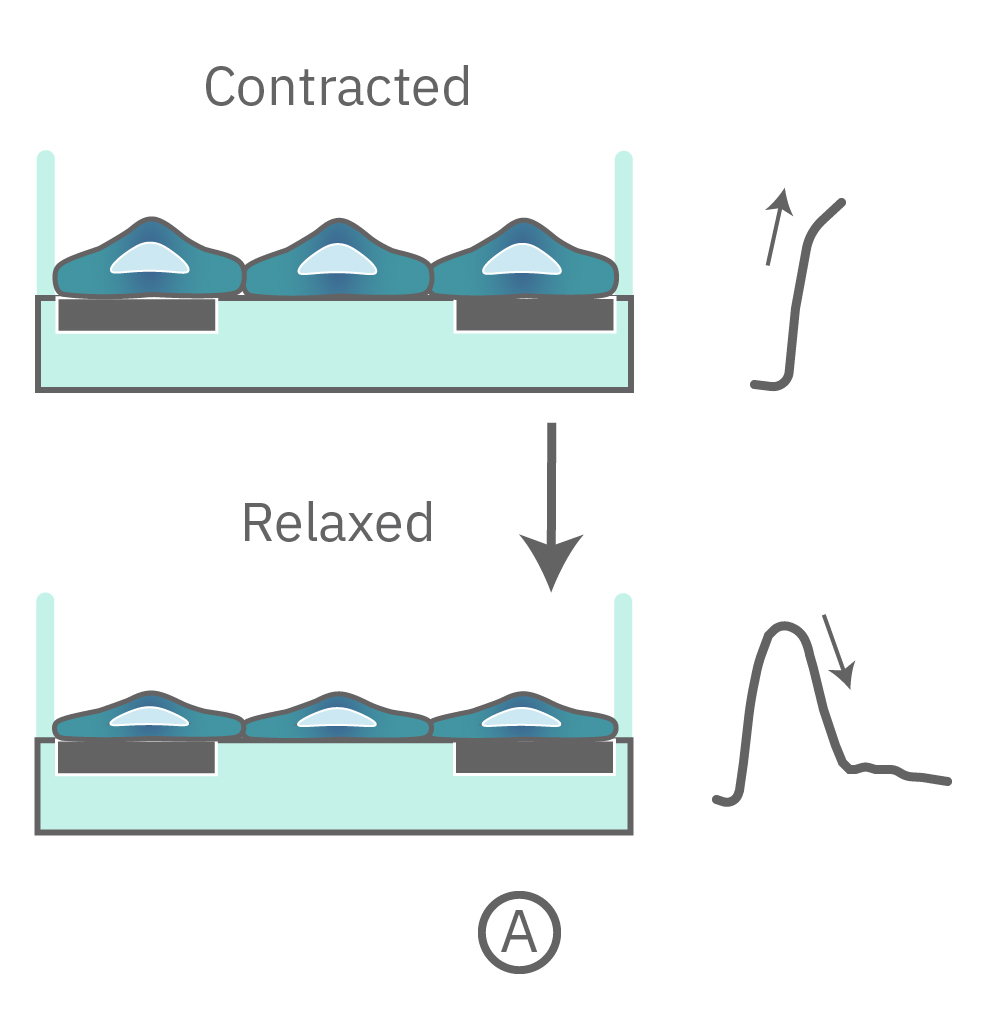
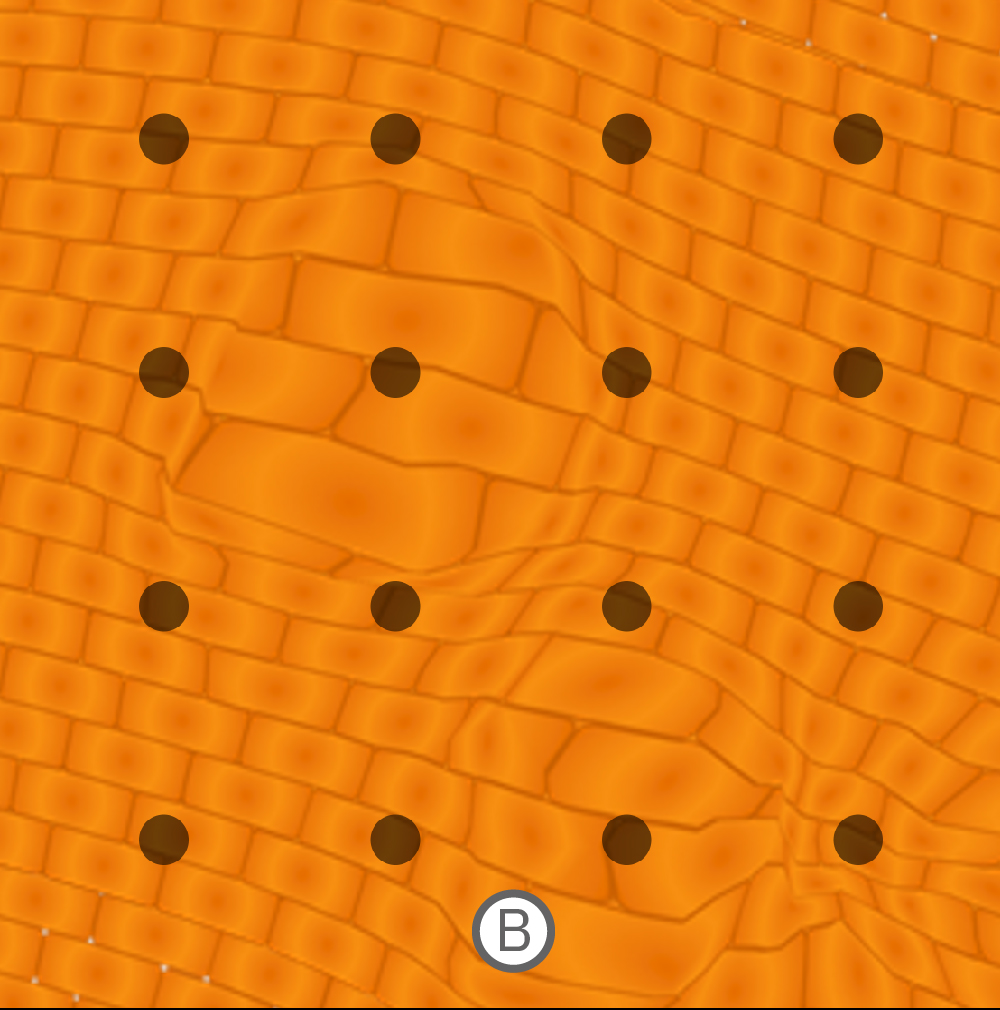
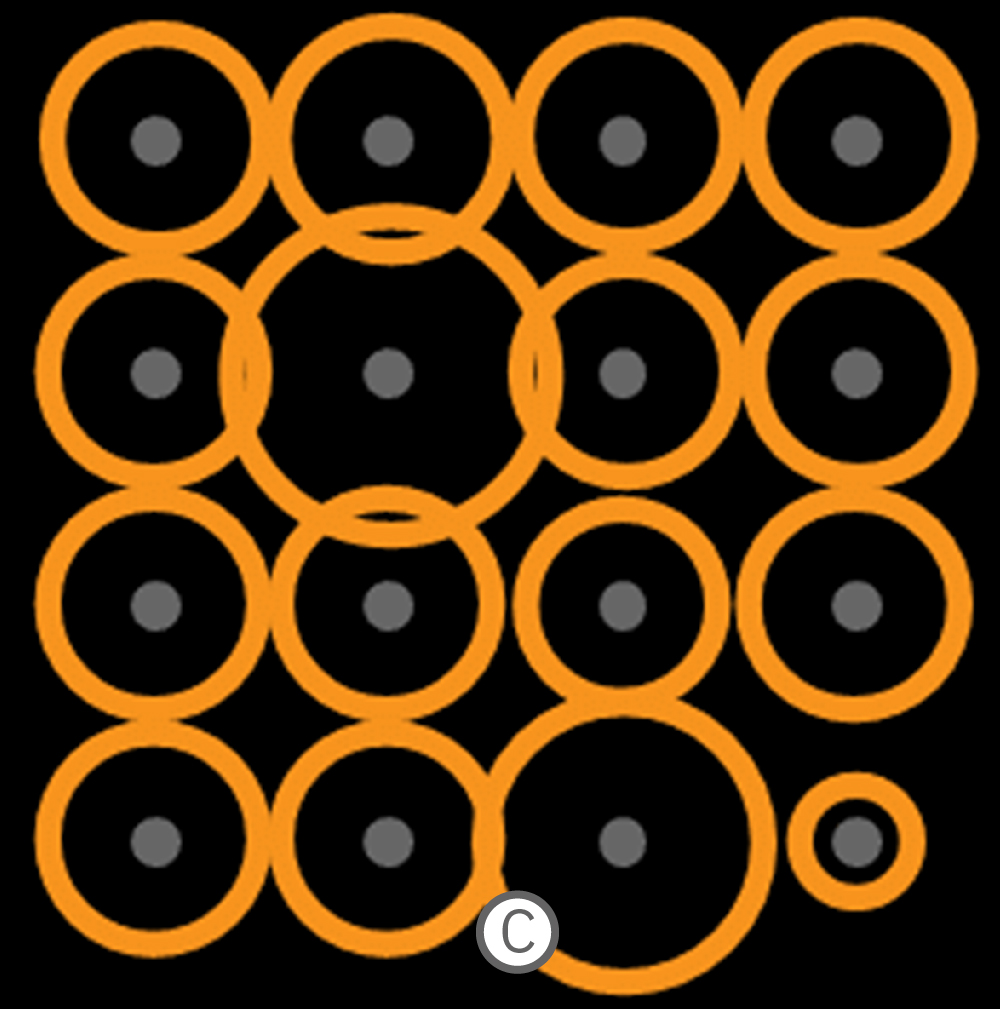
(A) Contraction and relaxation are detected as an increase and decrease in contractility (gray arrows). (B) The microelectrode array detects regions that are contracting while others are being stretched. (C) This pattern is represented as a contractility map, where the relative size of each circle indicates whether local cells are contracting or being stretched.
Contractility in 3D
Using an array of microelectrodes for contractility offers many advantages, including assessing variability across the syncytium and variations in cell culture coverage. Recording from microelectrodes enables advanced applications, such as measuring contractility from multiple 3D cardiac spheroids in the same well. A larger electrode would either smear these signals or fail to detect them at all. The microelectrodes provide high spatial resolution recordings to detect the distinct beating patterns in each area of the well.
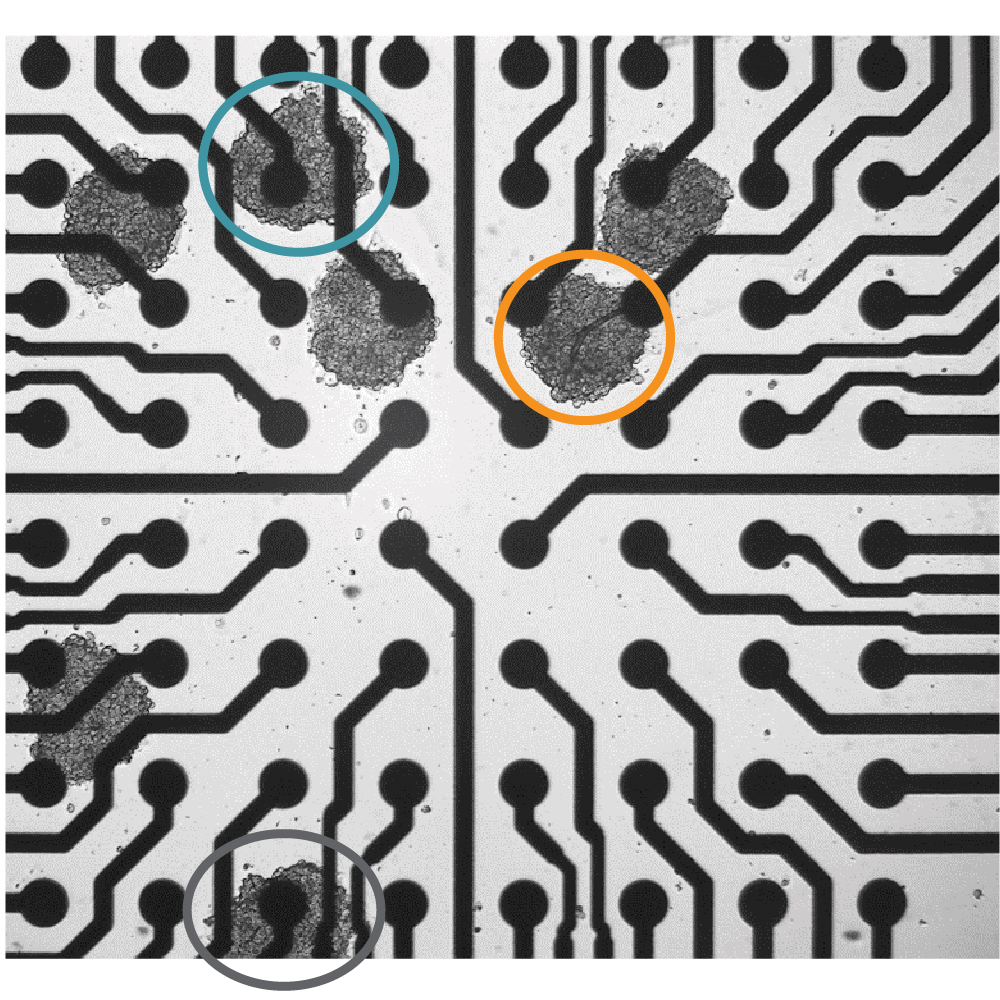
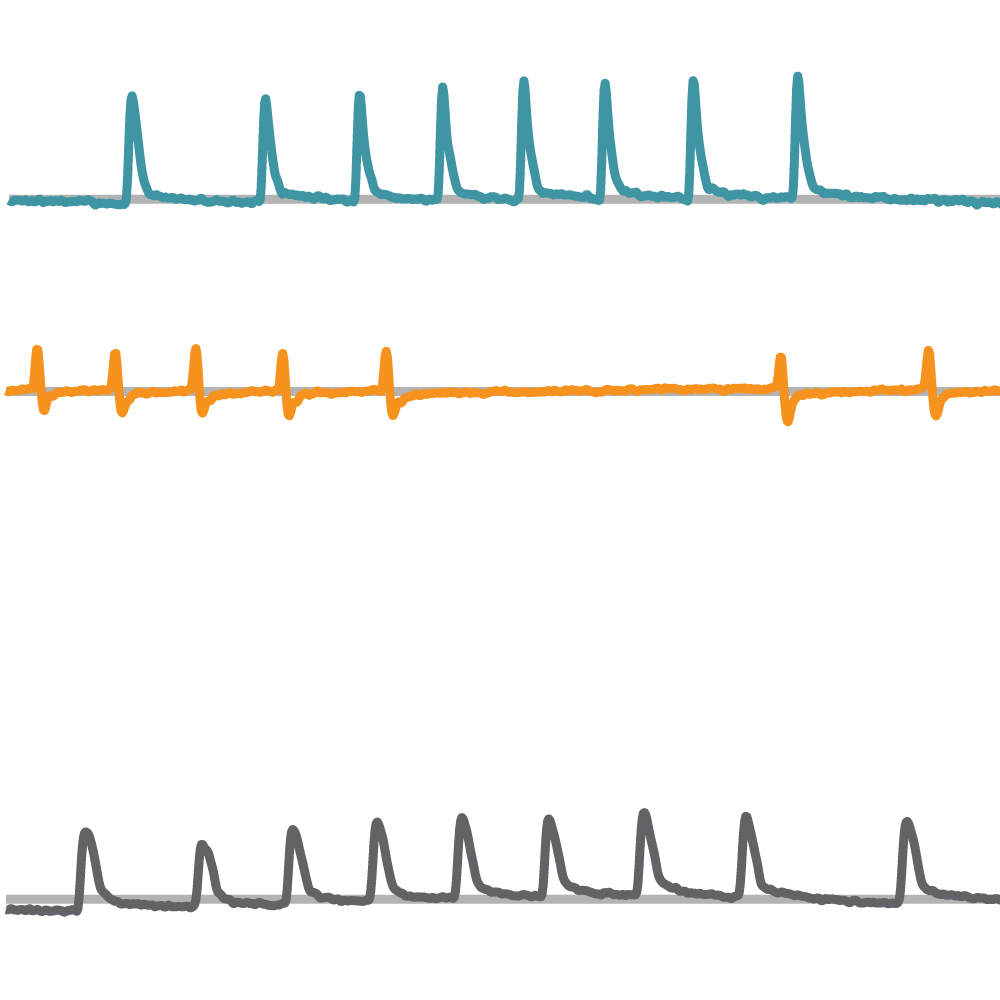
Seven spheroids of human induced pluripotent stem cell-derived cardiomyocytes were deposited in a CytoView MEA 6-well plate. The spheroids beat independently, exhibiting unique contractile patterns that were detected and distinguished using array-based contractility on the Maestro.
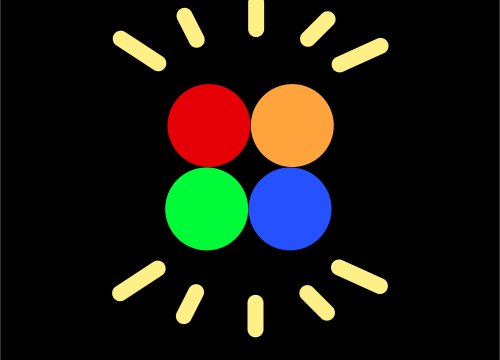
Optogenetics
Show Full DetailsOptogenetics: Using light to control cells
Optogenetics is a technique that involves the use of light to control cell function. Cells are first genetically modified to express light-sensitive ion channels, called opsins. Then, light can be used to activate the opsin. The most well-known opsins are light-gated ion channels that can control the excitability of the cell membrane. When activated by the opsin-specific wavelength of light, the channels open allowing ions to flow across the cell membrane to either excite or inhibit the cell. Optogenetics enables precise control over a targeted cell population.
Many opsins, many options
Since the first microbial opsin was introduced into mammalian neurons in 2005, many different opsins have been used to control the excitability of electroactive cells such as neurons and cardiomyocytes. Each opsin is sensitive to a specific wavelength range, or color of light and induces a precise biological event.
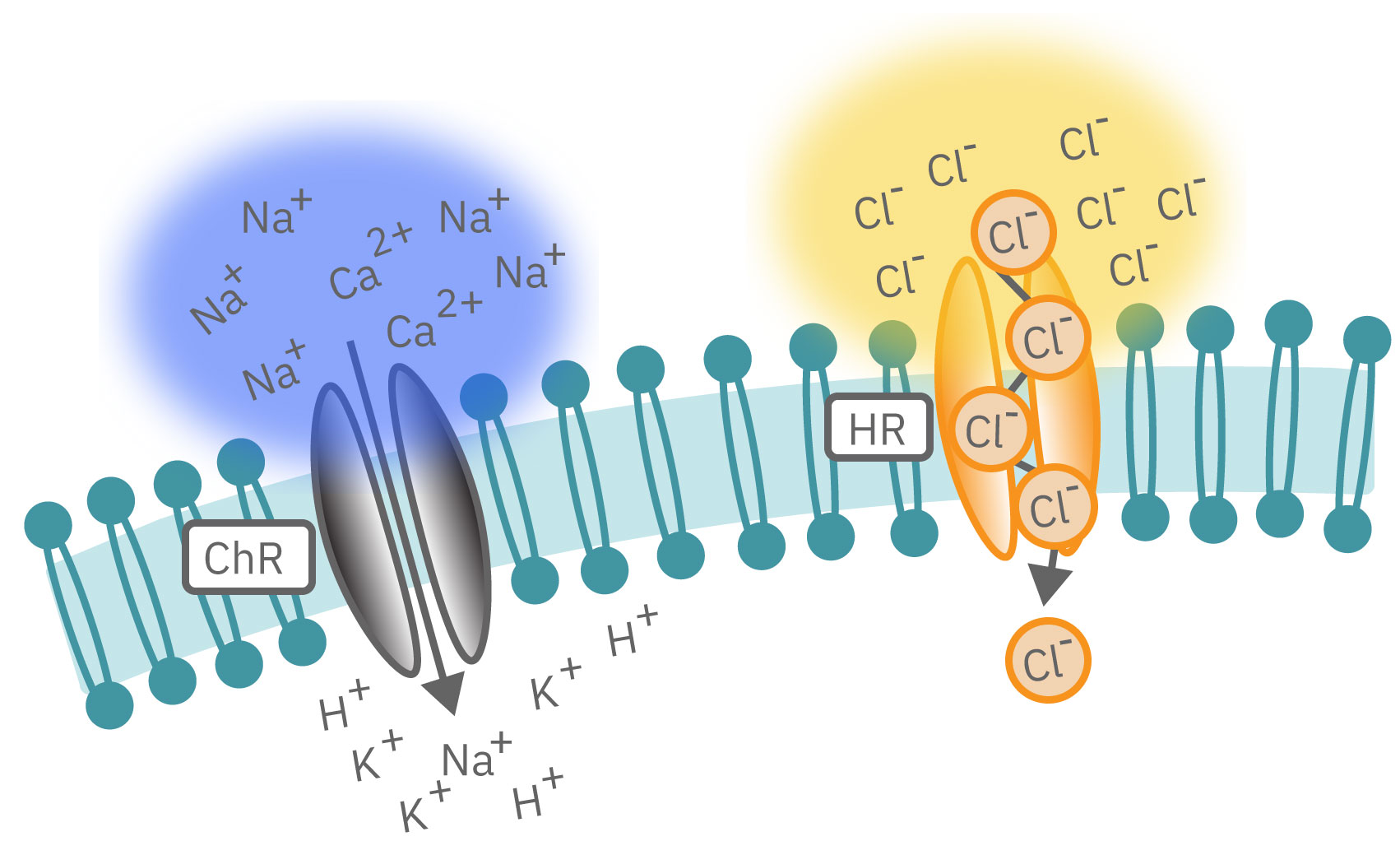
Channelrhodopsin (ChR2), for example, is activated by blue light. When ChR2 opens, positive cations (like sodium and calcium), flow into the cell, depolarizing or exciting the cell. In contrast, halorhodopsin and archaerhodopsin both inhibit cell excitability by hyperpolarizing the cell in response to yellow or green light, respectively. With optogenetics, you can turn on and off cells like a light switch.
The timing of these light-activated events is fast, facilitating highly precise control. First generation opsins, such as channelrhodopsin, open and close in milliseconds, ideal for kicking off an action potential. Second generation opsins have fined tuned kinetics for even faster, more precise control or slower, longer-lasting inhibition. For example, step-function opsins stay open until another pulse of light switches them off.
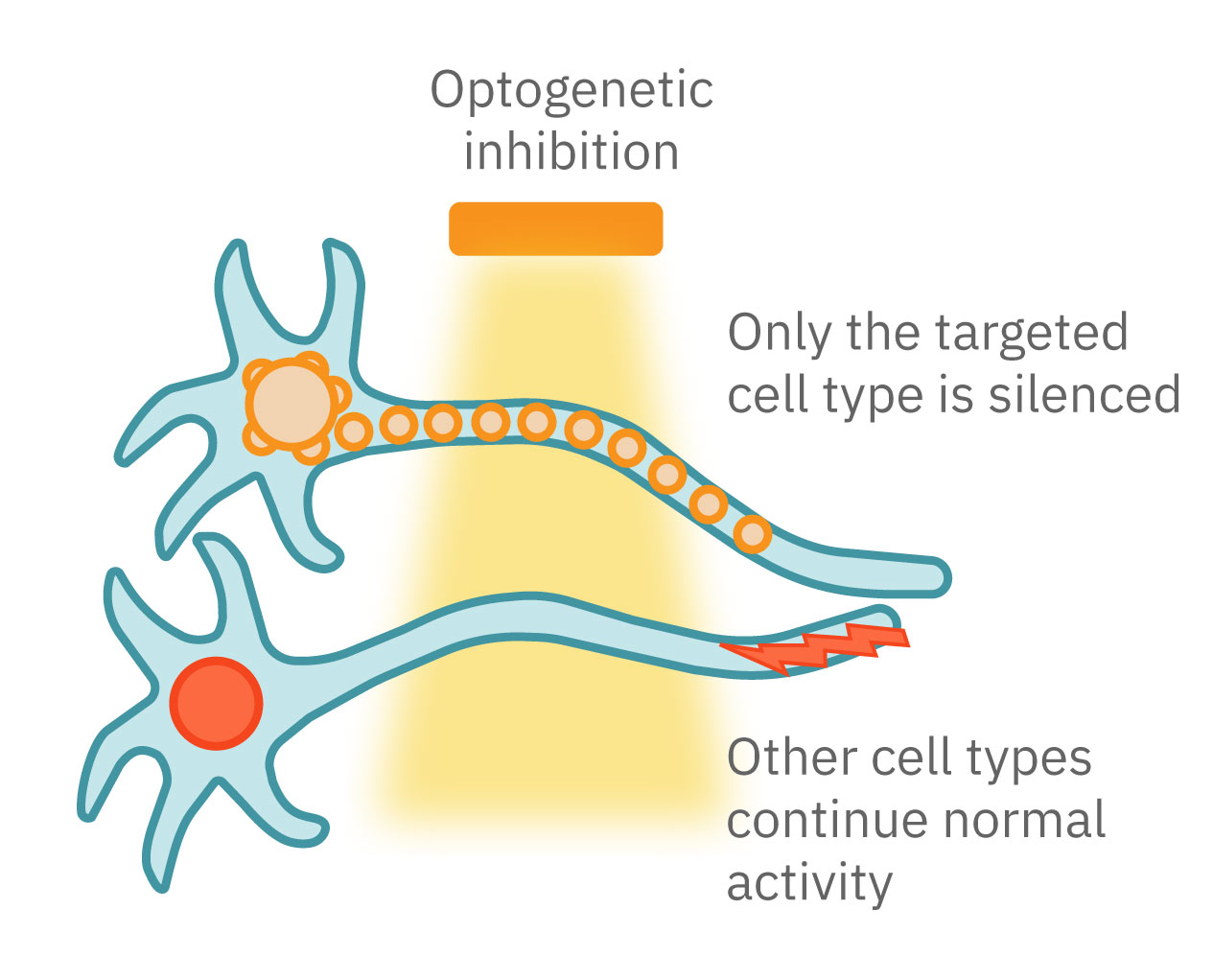
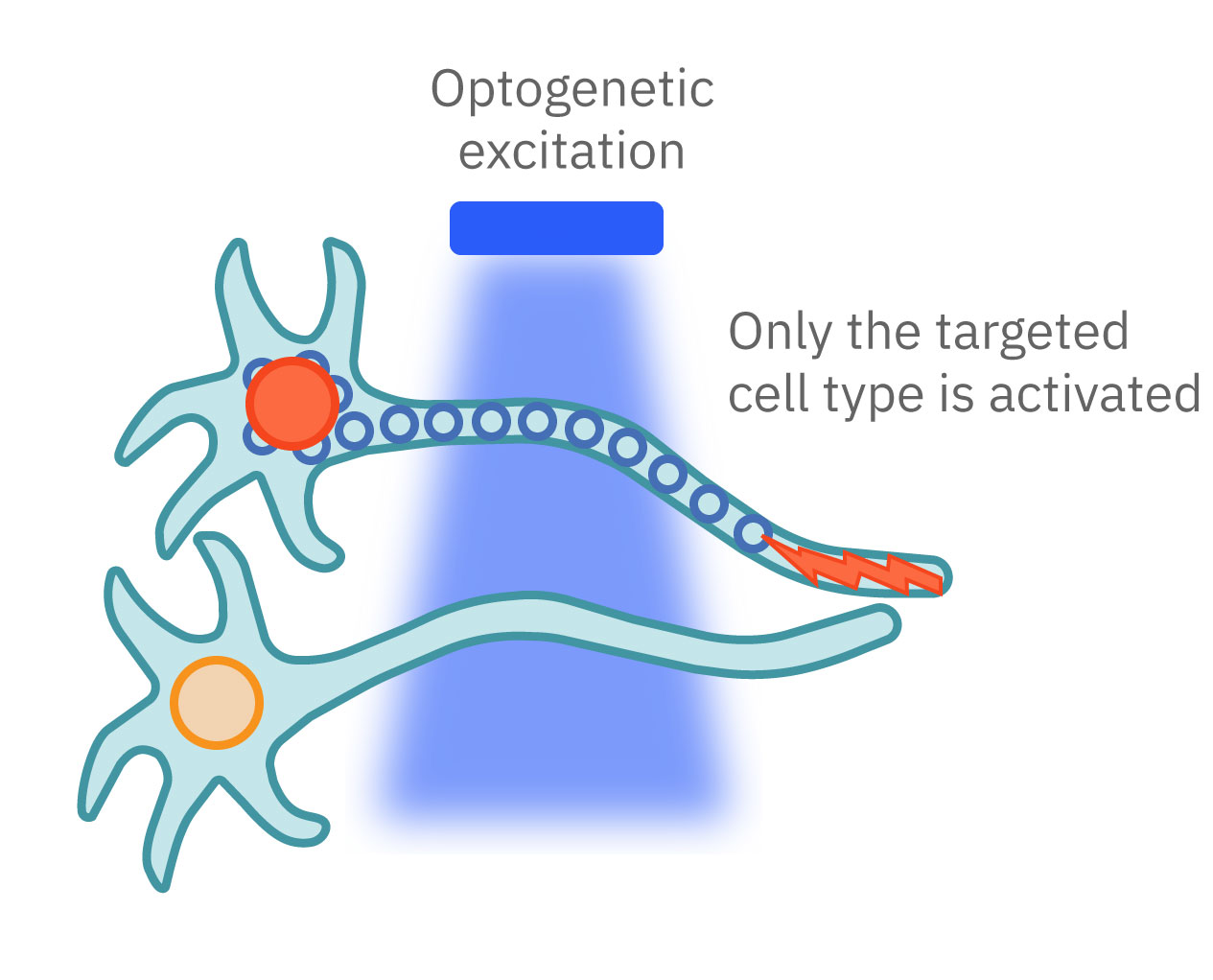
Optogenetics can precisely control which cells are turned on or off by employing different gene promoters for opsin expression. Opsins can be expressed in all neurons or used to control specific subpopulations. Unlike electrical stimulation, which excites all nearby cells, optical stimulation can be finely targeted to the cells expressing the opsins responsive to a narrow band of light wavelengths.
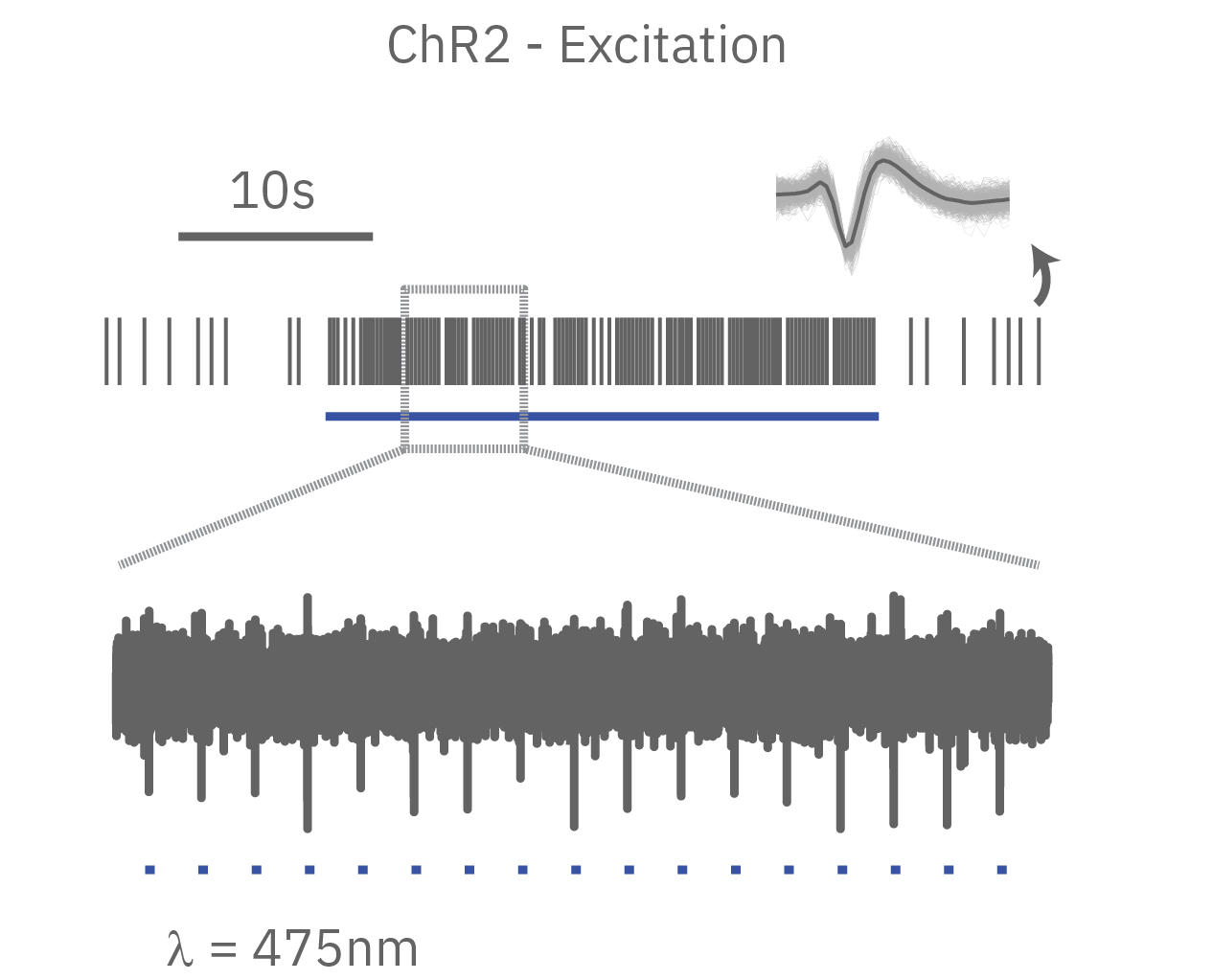
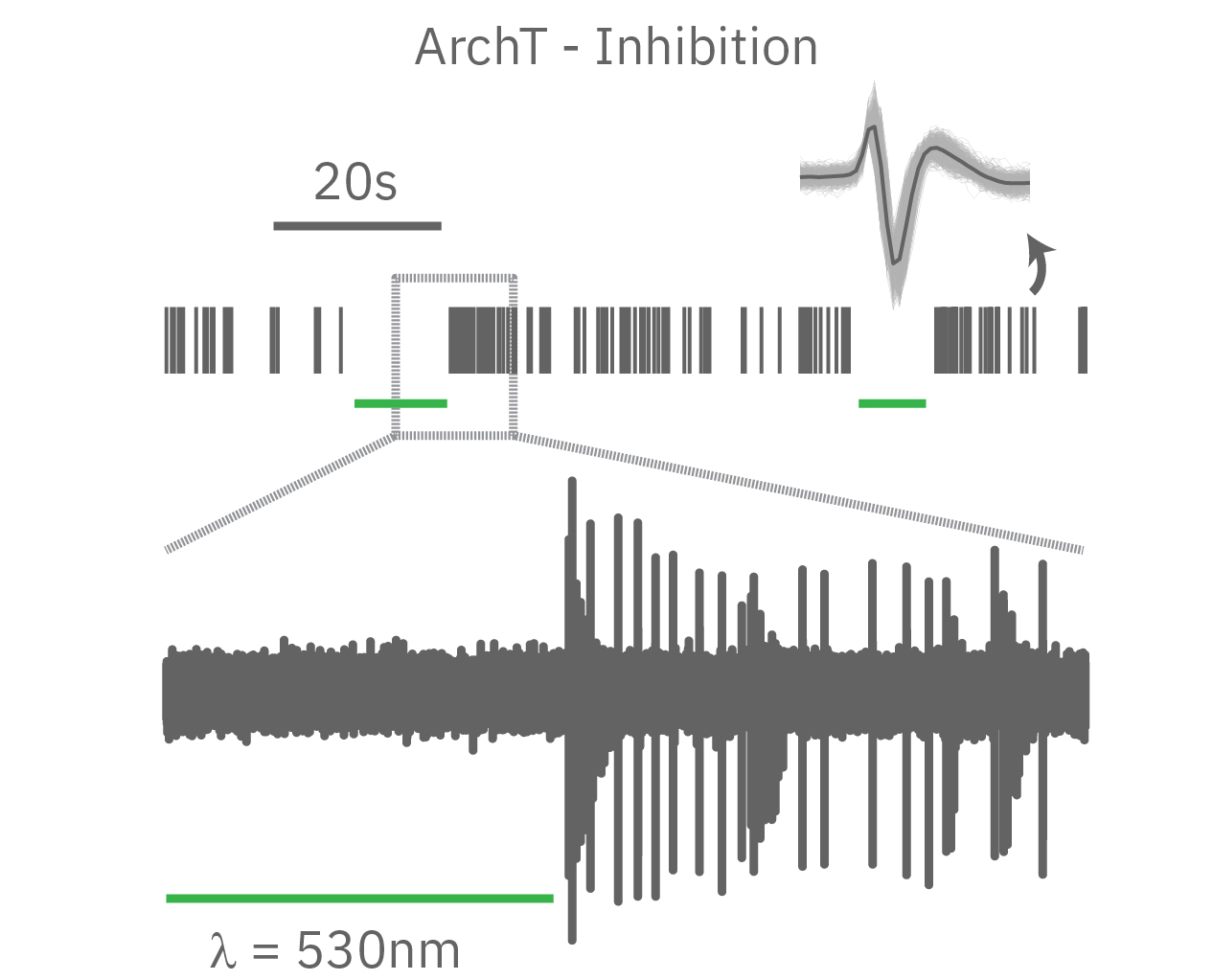
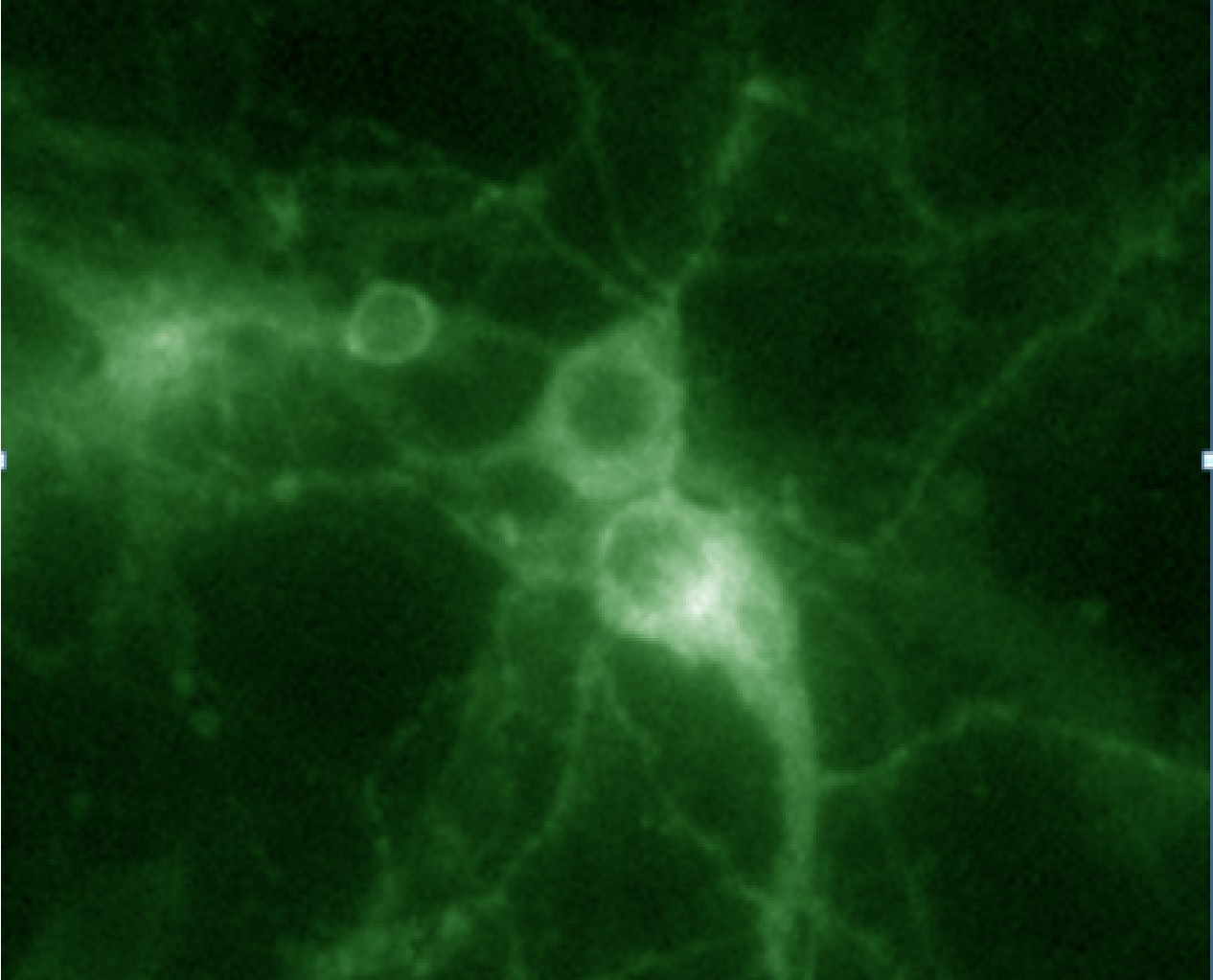
In summary, optogenetics is a powerful toolbox for precise control over targeted cell populations at fast time scales. Superior spatial and temporal control, reversibility, and easy stimulus delivery make exploring complex biology simpler than ever before.
More than just ion channels
As the field has advanced, opsins have been used to control more than just ion flow. Light-activated gene expression with light-inducible transcription factors can control the proteins made by cells. The combination of optogenetics with CRISPR provides even greater control over CRISPR/Cas9 gene editing.
Opsins have also been incorporated into many biochemical and intracellular signaling pathways to control key protein functions. MAPK and PI3K pathways, Rho family GTPase activation, apoptosis, and protein trafficking can now all be precisely controlled by light.
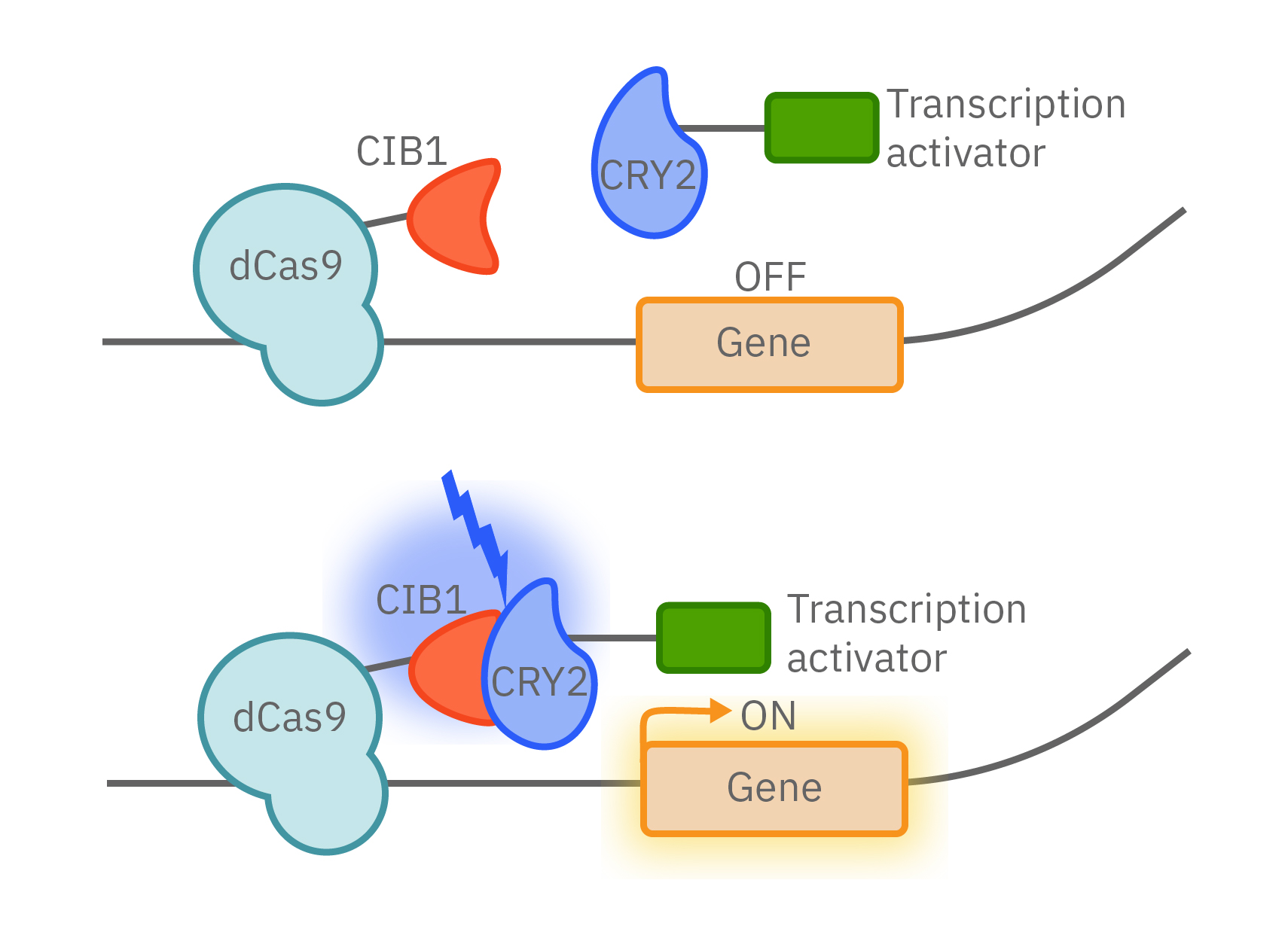
Shining light in vitro
Sophisticated biology like optogenetics demands sophisticated technology to explore it. In vitro technology relied on single wavelength lasers and custom lab-specific tools while many in vivo technologies were quickly developed for optical stimulation. The Lumos Optical Stimulation system is the first-of-its-kind multiwell optical stimulator with the ability to deliver up to four wavelengths of light per well with microsecond precision. From controlling the excitability of your neurons to pacing the beating of your cardiomyocytes, discover how the Lumos and optogenetics can revolutionize your assay.
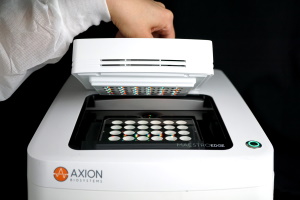